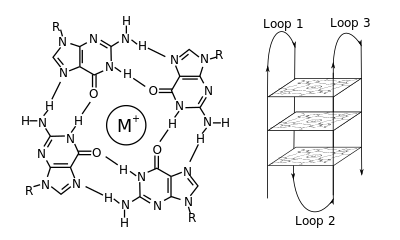
In molecular biology, G-quadruplex secondary structures (G4) are formed in nucleic acids by sequences that are rich in guanine. They are helical in shape and contain guanine tetrads that can form from one, two or four strands. The unimolecular forms often occur naturally near the ends of the chromosomes, better known as the telomeric regions, and in transcriptional regulatory regions of multiple genes, both in microbes and across vertebrates including oncogenes in humans. Four guanine bases can associate through Hoogsteen hydrogen bonding to form a square planar structure called a guanine tetrad (G-tetrad or G-quartet), and two or more guanine tetrads (from G-tracts, continuous runs of guanine) can stack on top of each other to form a G-quadruplex.
The placement and bonding to form G-quadruplexes is not random and serve very unusual functional purposes. The quadruplex structure is further stabilized by the presence of a cation, especially potassium, which sits in a central channel between each pair of tetrads. They can be formed of DNA, RNA, LNA, and PNA, and may be intramolecular, bimolecular, or tetramolecular. Depending on the direction of the strands or parts of a strand that form the tetrads, structures may be described as parallel or antiparallel. G-quadruplex structures can be computationally predicted from DNA or RNA sequence motifs, but their actual structures can be quite varied within and between the motifs, which can number over 100,000 per genome. Their activities in basic genetic processes are an active area of research in telomere, gene regulation, and functional genomics research.
History
The identification of structures with a high guanine association became apparent in the early 1960s, through the identification of gel-like substances associated with guanines. More specifically, this research detailed the four-stranded DNA structures with a high association of guanines, which was later identified in eukaryotic telomeric regions of DNA in the 1980s. The importance of discovering G-quadruplex structure was described through the statement, “If G-quadruplexes form so readily in vitro, Nature will have found a way of using them in vivo” - Aaron Klug, Nobel Prize Winner in Chemistry (1982). Interest in in vivo function of G-quadruplexes surged after large scale genome-wide analysis showed the prevalence of potential G-quadruplex (pG4)-forming sequences within gene promoters of human, chimpanzee, mouse, and rat - presented in the First International G-quadruplex Meeting held in April 2007 in Louisville, Kentucky. In 2006, the prevalence of G-quadruplexes within gene promoters of several bacterial genomes was reported predicting G-quadruplex-mediated gene regulation. With the abundance of G-quadruplexes in vivo, these structures hold a biologically relevant role through interactions with the promoter regions of oncogenes and the telomeric regions of DNA strands. Current research consists of identifying the biological function of these G-Quadruplex structures for specific oncogenes and discovering effective therapeutic treatments for cancer based on interactions with G-quadruplexes. Early evidence for the formation of G-quadruplexes in vivo in cells was established by isolating them from cells, and later by the observation that specific DNA helicases could be identified where small molecules specific for these DNA structures accumulated in cells.

Topology
The length of the nucleic acid sequences involved in tetrad formation determines how the quadruplex folds. Short sequences, consisting of only a single contiguous run of three or more guanine bases, require four individual strands to form a quadruplex. Such a quadruplex is described as tetramolecular, reflecting the requirement of four separate strands. The term G4 DNA was originally reserved for these tetramolecular structures that might play a role in meiosis. However, as currently used in molecular biology, the term G4 can mean G-quadruplexes of any molecularity. Longer sequences, which contain two contiguous runs of three or more guanine bases, where the guanine regions are separated by one or more bases, only require two such sequences to provide enough guanine bases to form a quadruplex. These structures, formed from two separate G-rich strands, are termed bimolecular quadruplexes. Finally, sequences which contain four distinct runs of guanine bases can form stable quadruplex structures by themselves, and a quadruplex formed entirely from a single strand is called an intramolecular quadruplex.
Depending on how the individual runs of guanine bases are arranged in a bimolecular or intramolecular quadruplex, a quadruplex can adopt one of a number of topologies with varying loop configurations. If all strands of DNA proceed in the same direction, the quadruplex is termed parallel. For intramolecular quadruplexes, this means that any loop regions present must be of the propeller type, positioned to the sides of the quadruplex. If one or more of the runs of guanine bases has a 5’-3’ direction opposite to the other runs of guanine bases, the quadruplex is said to have adopted an antiparallel topology. The loops joining runs of guanine bases in intramolecular antiparallel quadruplexes are either diagonal, joining two diagonally opposite runs of guanine bases, or lateral (edgewise) type loops, joining two adjacent runs of guanine base pairs.
In quadruplexes formed from double-stranded DNA, possible interstrand topologies have also been discussed . Interstrand quadruplexes contain guanines that originate from both strands of dsDNA.
Structure and functional role in genome
Following sequencing of the human genome, many guanine-rich sequences that had the potential to form quadruplexes were discovered. Depending on cell type and cell cycle, mediating factors such as DNA-binding proteins on chromatin, composed of DNA tightly wound around histone proteins, and other environmental conditions and stresses affect the dynamic formation of quadruplexes. For instance, quantitative assessments of the thermodynamics of molecular crowding indicate that the antiparallel g-quadruplex is stabilized by molecular crowding. This effect seems to be mediated by alteration of the hydration of the DNA and its effect on Hoogsteen base pair bonding. These quadruplexes seemed to readily occur at the ends of chromosome. In addition, the propensity of g-quadruplex formation during transcription in RNA sequences with the potential to form mutually exclusive hairpin or G-quadruplex structures depends heavily on the position of the hairpin-forming sequence.
Because repair enzymes would naturally recognize ends of linear chromosomes as damaged DNA and would process them as such to harmful effect for the cell, clear signaling and tight regulation is needed at the ends of linear chromosomes. Telomeres function to provide this signaling. Telomeres, rich in guanine and with a propensity to form g-quadruplexes, are located at the terminal ends of chromosomes and help maintain genome integrity by protecting these vulnerable terminal ends from instability.
These telomeric regions are characterized by long regions of double-stranded CCCTAA:TTAGGG repeats. The repeats end with a 3’ protrusion of between 10 and 50 single-stranded TTAGGG repeats. The heterodimeric complex ribonucleoprotein enzyme telomerase adds TTAGGG repeats at the 3’ end of DNA strands. At these 3’ end protrusions, the G-rich overhang can form secondary structures such as G-quadruplexes if the overhang is longer than four TTAGGG repeats. The presence of these structures prevent telomere elongation by the telomerase complex.
Telomeric quadruplexes
Telomeric repeats in a variety of organisms have been shown to form these quadruplex structures in vitro, and subsequently they have also been shown to form in vivo. The human telomeric repeat (which is the same for all vertebrates) consists of many repeats of the sequenced (TTAGGG), and the quadruplexes formed by this structure can be in bead-like structures of 5 nm to 8 nm in size and have been well studied by NMR, TEM and X-ray crystal structure determination. The formation of these quadruplexes in telomeres has been shown to decrease the activity of the enzyme telomerase, which is responsible for maintaining length of telomeres and is involved in around 85% of all cancers. This is an active target of drug discovery, including telomestatin.
Non-telomeric quadruplexes
Quadruplexes are present in locations other than at the telomere. Analysis of human, chimpanzee, mouse and rat genomes showed enormous number of potential G-quadruplex (pG4)-forming sequences in non-telomeric regions. A large number of the non-telomeric G-quadruplexes were found within gene promoters, and were conserved across the species. Similarly, large number of G-quadruplexes were found in the E. coli and hundreds of other microbial genomes. Here also, like vertebrates G-quadruplexes were enriched within gene promoters. In addition, there was found more than one-billion-year conserved G-quadruplex locus in plants and algae, in gene encoding large subunit of RNA polymerase II. Although these studies predicted G-quadruplex-mediated gene regulation, it is unlikely that all pG4s would form in vivo. The proto-oncogene c-myc forms a quadruplex in a nuclease hypersensitive region critical for gene activity. Other genes shown to form G-quadruplexes in their promoter regions include the chicken β-globin gene, human ubiquitin-ligase RFP2, and the proto-oncogenes c-kit, bcl-2, VEGF, H-ras and N-ras.
Genome-wide surveys based on a quadruplex folding rule have been performed, which have identified 376,000 Putative Quadruplex Sequences (PQS) in the human genome, although not all of these probably form in vivo. A similar studies have identified putative G-quadruplexes in prokaryotes, namely the bacterium E. coli. There are several possible models for how quadruplexes could influence gene activity, either by upregulation or downregulation. One model is shown below, with G-quadruplex formation in or near a promoter blocking transcription of the gene, and hence de-activating it. In another model, quadruplex formed at the non-coding DNA strand helps to maintain an open conformation of the coding DNA strand and enhance an expression of the respective gene.
Function
It has been suggested that quadruplex formation plays a role in immunoglobulin heavy chain switching. As cells have evolved mechanisms for resolving (i.e., unwinding) quadruplexes that form. Quadruplex formation may be potentially damaging for a cell; the helicases WRN and Bloom syndrome protein have a high affinity for resolving DNA G-quadruplexes. The DEAH/RHA helicase, DHX36, has also been identified as a key G-quadruplex resolvase. In 2009, a metastasis suppressor protein NM23H2 (also known as NME2) was found to directly interact with G-quadruplex in the promoter of the c-myc gene, and transcriptionally regulate c-myc. More recently, NM23H2 was reported to interact with G-quadruplex in the promoter of the human telomerase (hTERT) gene and regulate hTERT expression In 2019, the telomere-binding-factor-2 (TRF2 or TERF2) was shown to bind to thousands of non-telomeric G-quadruplexes in the human genome by TRF2 ChIP-seq. There are many studies that implicate quadruplexes in both positive and negative transcriptional regulation, including epigenetic regulation of genes like hTERT. Function of G-quadruplexes have also been reported in allowing programmed recombination of immunologlobin heavy genes and the pilin antigenic variation system of the pathogenic Neisseria. The roles of quadruplex structure in translation control are not as well explored. The direct visualization of G-quadruplex structures in human cells as well as the co-crystal structure of an RNA helicase bound to a G-quadruplex have provided important confirmations of their relevance to cell biology. The potential positive and negative roles of quadruplexes in telomere replication and function remains controversial. T-loops and G-quadruplexes are described as the two tertiary DNA structures that protect telomere ends and regulate telomere length.
Genome Regulation through formation of G-quadruplex structures
Many of the genome regulatory processes have been linked to the formation of G-quadruplex structures, attributable to the huge role it plays in DNA repair of apurinic/apyrimidinic sites also known as AP sites. A new technique to map AP sites has been developed known as AP-seq which utilizes a biotin-labeled aldehyde-reactive probe (ARP) to tag certain regions of the genome where AP site damage occurrence has been significant. Another genome-wide mapping sequencing method known as ChIP-sequencing, was utilized to map both; damage in AP sites, and the enzyme responsible for its repair, AP endonuclease 1 (APE1). Both of these genome-wide mapping sequencing methods, ChIP-sequencing and ARP, have indicated that AP site damage occurrence is nonrandom. AP site damage was also more prevalent in certain regions of the genome that contain specific active promoter and enhancer markers, some of which were linked to regions responsible for lung adenocarcinoma and colon cancer. AP site damage was found to be predominant in PQS regions of the genome, where formation of G-quadruplex structures is regulated and promoted by the DNA repair process, base excision repair (BER). Base excision repair processes in cells have been proved to be reduced with aging as its components in the mitochondria begin to decline, which can lead to the formation of many diseases such as Alzheimer's disease (AD). These G-quadruplex structures are said to be formed in the promoter regions of DNA through superhelicity, which favors the unwinding of the double helical structure of DNA and in turn loops the strands to form G-quadruplex structures in guanine rich regions. The BER pathway is signalled when it indicates an oxidative DNA base damage, where structures like, 8-Oxoguanine-DNA glycosylase 1 (OGG1), APE1 and G-quadruplex play a huge role in its repair. These enzymes participate in BER to repair certain DNA lesions such as 7,8-dihydro-8-oxoguanine (8-oxoG), which forms under oxidative stress to guanine bases.
Role of Endogenous Oxidized DNA Base Damage on G4 formation
Guanine (G) bases in G-quadruplex have the lowest redox potential causing it to be more susceptible to the formation of 8-oxoguanine (8-oxoG), an endogenous oxidized DNA base damage in the genome. Due to Guanine having a lower electron reduction potential than the other nucleotides bases,8-oxo-2'-deoxyguanosine (8-oxo-dG), is a known major product of DNA oxidation. Its concentration is used as a measurement of oxidative stress within a cell. When DNA undergoes oxidative damage, a possible structural change in guanine, after ionizing radiation, gives rise to an enol form, 8-OH-Gua. This oxidative product is formed through a tautomeric shift from the original damage guanine, 8-oxo-Gua, and represents DNA damage that causes changes in the structure. This form allows for the base excision repair (BER) enzyme OGG1 to bind and remove the oxidative damage with the help of APE1, resulting in an AP site. Moreover, an AP site is a location in DNA that has neither a purine or a pyrimidine base due to DNA damage, they are the most prevalent type of endogenous DNA damage in cells. AP sites can be generated spontaneously or after the cleavage of modified bases, like 8-OH-Gua. The generation of an AP site enables the melting of the duplex DNA to unmask the PQS, adopting a G-quadruplex fold. With the use of genome-wide ChIP-sequencing analyses, cell-based assays, and in vitro biochemical analyses, a connection has been made between oxidized DNA base-derived AP sites, and the formation of the G-quadruplex.
DNA Oxidation Contribution to Diseases
Furthermore, the concentration of 8-oxo-dG is a known biomarker of oxidative stress within a cell, and excessive amount of oxidative stress has been linked to carcinogenesis and other diseases. When produced, 8-oxo-dG, has the ability to inactivate OGG1, thus preventing the repair of DNA damage caused by the oxidation of guanine. The possible inactivation allows for un-repaired DNA damages to gather in non-replicating cells, like muscle, and can cause aging as well. Moreover, oxidative DNA damage like 8-oxo-dG contributes to carcinogenesis through the modulation of gene expression, or the induction of mutations. On the condition that 8-oxo-dG is repaired by BER, parts of the repair protein is left behind which can lead to epigenetic alterations, or the modulation of gene expression. Upon insertion of 8-oxo-dG into thymidine kinase gene of humans, it was determine that if 8-oxo-dG was left unchecked and not repaired by BER, it can lead to frequent mutations and eventually carcinogenesis.
APE1 role in Gene Regulation
AP endonuclease 1 (APE1) is an enzyme responsible for the promotion and the formation of G-quadruplex structures. APE1 is mainly in charge of repairing damage caused to AP sites through the BER pathway. APE1 is considered to be very crucial as AP site damage is known to be the most recurring type of endogenous damage to DNA. The oxidation of certain purine bases, like guanine, forms oxidized nucleotides that impairs DNA function by mismatching nucleotides in the sequences. This is more common in PQS sequences which form oxidized structures, such as 8-oxoguanine. Once the cell is aware of oxidative stress and damage, it recruits OGG1 to the site, whose main function is to initiate the BER pathway. OGG1 does so by cleaving the oxidized base and thus creating an AP site, primarily through the process of negative superhelicity. This AP site then signals cells to engage APE1 binding, which binds to the open duplex region. The binding of APE1 then plays an important role by stabilizing the formation of G-quadruplex structures in that region. This promotes formation of G-quadruplex structures by the folding of the stand. This looping process brings four bases in close proximity that will be held together by Hoogsteen base pairing. After this stage the APE1 gets acetylated by multiple lysine residues on the chromatin, forming acetylated APE1 (AcAPE1). AcAPE1 is very crucial to the BER pathway as it acts as a transcriptional coactivator or corepressor, functioning to load transcription factors (TF) into the site of damage allowing it to regulate the gene expression. AcAPE1 is also very important since it allows APE1 to bind for longer periods of time by delay of its dissociation from the sequence, allowing the repair process to be more efficient. Deacetylation of AcAPE1 is the driving force behind the loading of these TFs, where APE1 dissociates from the G-quadruplex structures. When a study downregulated the presence of APE1 and AcAPE1 in the cell, the formation of G-quadruplex structures was inhibited, which proves the importance of APE1 for the formation of these structures. However, not all G-quadruplex structures require APE1 for formation, in fact some of them formed greater G-quadruplex structures in its absence. Therefore, we can conclude that APE1 has two important roles in genome regulation- Stabilizing the formation of g-quadruplex structures and loading the transcriptional factors onto the AP site
Cancer
Telomeres
G-quadruplex forming sequences are prevalent in eukaryotic cells, especially in telomeres, 5` untranslated strands, and translocation hot spots. G-quadruplexes can inhibit normal cell function, and in healthy cells, are easily and readily unwound by helicase. However, in cancer cells that have mutated helicase these complexes cannot be unwound and leads to potential damage of the cell. This causes replication of damaged and cancerous cells. For therapeutic advances, stabilizing the G-quadruplexes of cancerous cells can inhibit cell growth and replication leading to the cell's death.
Promoter Regions
Along with the association of G-quadruplexes in telomeric regions of DNA, G-quadruplex structures have been identified in various human proto-oncogene promoter regions. The structures most present in the promoter regions of these oncogenes tend to be parallel-stranded G-quadruplex DNA structures. Some of these oncogenes include c-KIT, PDGF-A, c-Myc and VEGF, showing the importance of this secondary structure in cancer growth and development. While the formation of G-quadruplex structure vary to some extent for the different promoter regions of oncogenes, the consistent stabilization of these structures have been found in cancer development. Current therapeutic research actively focuses on targeting this stabilization of G-quadruplex structures to arrest unregulated cell growth and division.
One particular gene region, the c-myc pathway, plays an integral role in the regulation of a protein product, c-Myc. With this product, the c-Myc protein functions in the processes of apoptosis and cell growth or development and as a transcriptional control on human telomerase reverse transcriptase. Interaction of c-Myc promoter G-quadruplex with NM23H2 was shown to regulate c-Myc in cancer cells in 2009
Regulation of c-myc through Human telomerase reverse transcriptase (hTERT) is also directly regulated through promoter G-quadruplex by interaction with the transcription factor NM23H2 where epigenetic modifications were dependent on NM23H2-G-quadruplex association. Recently, hTERT epigenetic regulation reported to be mediated through interaction of hTERT promoter G-quadruplex with the telomeric factor TRF2.
Another gene pathway deals with the VEGF gene, Vascular Endothelial Growth Factor, which remains involved in the process of angiogenesis or the formation of new blood vessels. The formation of an intramolecular G-quadruplex structure has been shown through studies on the polypurine tract of the promoter region of the VEGF gene. Through recent research on the role of G-quadruplex function in vivo, the stabilization of G-quadruplex structures was shown to regulate VEGF gene transcription, with inhibition of transcription factors in this pathway. The intramolecular G-quadruplex structures are formed mostly through the abundant guanine sequence in the promoter region of this specific pathway. The cyclin-dependent cell cycle checkpoint kinase inhibitor-1 CDKN1A (also known as p21) gene harbours promoter G-quadruplex. Interaction of this G-quadruplex with TRF2 (also known as TERF2) resulted in epigenetic regulation of p21, which was tested using the G-quadruplex-binding ligand 360A.
Hypoxia inducible factor 1ɑ, HIF-1ɑ, remains involved in cancer signaling through its binding to Hypoxia Response Element, HRE, in the presence of hypoxia to begin the process of angiogenesis. Through recent research into this specific gene pathway, the polypurine and polypyrimidine region allows for the transcription of this specific gene and the formation of an intramolecular G-quadruplex structure. However, more research is necessary to determine whether the formation of G-quadruplex regulates the expression of this gene in a positive or negative manner.
The c-kit oncogene deals with a pathway that encodes an RTK, which was shown to have elevated expression levels in certain types of cancer. The rich guanine sequence of this promoter region has shown the ability to form a variety of quadruplexes. Current research on this pathway is focusing on discovering the biological function of this specific quadruplex formation on the c-kit pathway, while this quadruplex sequence has been noticed in various species.
The RET oncogene functions in the transcription of kinase which has been abundant in certain types of cancer. The guanine rich sequence in the promoter region for this pathway exudes a necessity for baseline transcription of this receptor tyrosine kinase. In certain types of cancers, the RET protein has shown increased expression levels. The research on this pathway suggested the formation of a G-quadruplex in the promoter region and an applicable target for therapeutic treatments.
Another oncogene pathway involving PDGF-A, platelet-derived growth factor, involves the process of wound healing and function as mitogenic growth factors for cells. High levels of expression of PDGF have been associated with increased cell growth and cancer. The presence of a guanine-rich sequence in the promoter region of PDGF-A has exhibited the ability to form intramolecular parallel G-quadruplex structures and remains suggested to play a role in transcriptional regulation of PDGF-A. However, research has also identified the presence of G-quadruplex structures within this region due to the interaction of TMPyP4 with this promoter sequence.
Therapeutics
Telomeres are generally made up of G-quadruplexes and remain important targets for therapeutic research and discoveries. These complexes have a high affinity for porphyrin rings which makes them effective anticancer agents. However, TMPyP4 has been limited for used due to its non-selectivity toward cancer cell telomeres and normal double stranded DNA (dsDNA). To address this issue analog of TMPyP4, it was synthesized known as 5Me which targets only G quadruplex DNA which inhibits cancer growth more effectively than TMPyP4.
Ligand design and development remains an important field of research into therapeutic reagents due to the abundance of G-quadruplexes and their multiple conformational differences. One type of ligand involving a Quindoline derivative, SYUIQ-05, utilizes the stabilization of G-quadruplexes in promoter regions to inhibit the production of both the c-Myc protein product and the human telomerase reverse transcriptase (hTERT). This main pathway of targeting this region results in the lack of telomerase elongation, leading to arrested cell development. Further research remains necessary for the discovery of a single gene target to minimize unwanted reactivity with more efficient antitumor activity.
Ligands which bind quadruplexes
One way of inducing or stabilizing G-quadruplex formation is to introduce a molecule which can bind to the G-quadruplex structure. A number of ligands, which can be both small molecules and proteins, can bind to the G-quadruplex. These ligands can be naturally occurring or synthetic. This has become an increasingly large field of research in genetics, biochemistry, and pharmacology.
Cationic porphyrins have been shown to bind intercalatively with G-quadruplexes, as well as the molecule telomestatin.
The binding of ligands to G-quadruplexes is vital for anti-cancer pursuits because G-quadruplexes are found typically at translocation hot spots. MM41, a ligand that binds selectively for a quadruplex on the BCL-2 promoter, is shaped with a central core and 4 side chains branching sterically out. The shape of the ligand is vital because it closely matches the quadruplex which has stacked quartets and the loops of nucleic acids holding it together. When bound, MM41's central chromophore is situated on top of the 3’ terminal G-quartet and the side chains of the ligand associate to the loops of the quadruplex. The quartet and the chromophore are bound with a π-π bond while the side chains and loops are not bound but are in close proximity. What makes this binding strong is the fluidity in the position of the loops to better associate with the ligand side chains.
TMPyP4, a cationic porphyrin, is a more well known G4 binding ligand that helps to repress c-Myc. The way in which TMPyP4 binds to G4's is similar to MM41, with the ring stacking onto the external G-quartet and side chains associating to the loops of G4's.
When designing ligands to be bound to G-quadruplexes, the ligands have a higher affinity for parallel folded G-quadruplexes. It has been found that ligands with smaller side chains bind better to the quadruplex because smaller ligands have more concentrated electron density. Furthermore, the hydrogen bonds of ligands with smaller side chains are shorter and therefore stronger. Ligands with mobile side chains, ones that are able to rotate around its center chromophore, associate more strongly to G-quadruplexes because conformation of the G4 loops and the ligand side chains can align.
Quadruplex prediction techniques
Identifying and predicting sequences which have the capacity to form quadruplexes is an important tool in further understanding their role. Generally, a simple pattern match is used for searching for possible intrastrand quadruplex forming sequences: d(G3+N1-7G3+N1-7G3+N1-7G3+), where N is any nucleotide base (including guanine). This rule has been widely used in on-line algorithms. Although the rule effectively identifies sites of G-quadruplex formation, it also identifies a subset of the imperfect homopurine mirror repeats capable of triplex formation and C-strand i-motif formation. Moreover, these sequences also have the capacity to form slipped and foldback structures that are implicit intermediates in the formation of both quadruplex and triplex DNA structures. In one study, it was found that the observed number per base pair (i.e. the frequency) of these motifs has increased rapidly in the eumetazoa for which complete genomic sequences are available. This suggests that the sequences may be under positive selection enabled by the evolution of systems capable of suppressing non-B structure formation.
More recently, advanced web-based toolboxes for identifying G-quadruplex forming sequences were developed, including user-friendly and open access version of G4Hunter based on sliding window approach or G4RNA Screener based on machine learning algorithm.
Methods for studying G-quadruplexes
A number of experimental methods have been developed to identify G-quadruplexes. These methods can be broadly defined into two classes: biophysical and biochemical methods.
Biochemical methods
Biochemical techniques were employed to interrogate G-quadruplex formation in a longer sequence context. In the DNA polymerase stop assay, the formation of a G-quadruplex in a DNA template can act as a roadblock and cause polymerase stalling, which halts the primer extension. The dimethyl sulfate (DMS) followed by the piperidine cleavage assay is based on the fact that the formation of a G-quadruplex will prohibit the N7 guanine methylation caused by DMS, leading to a protection pattern observed at the DNA G-quadruplex region after piperidine cleavage.
Biophysical methods
The topology of the G-quadruplex structure can be determined by monitoring the positive or negative circular dichroism (CD) signals at specific wavelengths. Parallel G-quadruplexes have negative and positive CD signals at 240 and 262 nm, respectively, whereas antiparallel G-quadruplexes place these signals at 262 and 295 nm, respectively. To verify G-quadruplex formation, one should also perform the CD experiments under non-G-quadruplex stabilizing (Li+) and G-quadruplex stabilizing conditions (such as K+ or with G-quadruplex ligands), and scan toward the far-UV region (180–230 nm).
The thermostability of the G-quadruplex structure can be identified by observing the UV signal at 295 nm. Upon G-quadruplex melting, the UV absorbance at 295 nm decreases, leading to a hypochromic shift that is a distinctive feature of G-quadruplex structure.
As the quantum yields of the intrinsic G-quadruplex fluorescence are higher compared to those of the constitutive single strands, their formation or their melting can be detected by monitoring their emission. The intensity changes obseved upon excitation at 295 nm (see above) renders this method particularly sensitive.
Another approach for detection of G-quadruplexes includes nanopore-based methods. Firstly, it was shown that biological nanopores can detect G-quadruplexes based on size exclusion and specific interaction of G-quadruplex and protein nanocavity. The novel approach combines solid-state nanopores and DNA nanotechnology for label-free detection of G-quadruplexes, for their mapping on dsDNA, and for monitoring G-quadruplex formation.
Role in neurological disorders
G-quadruplexes have been implicated in neurological disorders through two main mechanisms. The first is through expansions of G-repeats within genes that lead to the formation of G-quadruplex structures that directly cause disease, as is the case with the C9orf72 gene and amyotrophic lateral sclerosis (ALS) or frontotemporal dementia (FTD). The second mechanism is through mutations that affect the expression of G-quadruplex binding proteins, as seen in the fragile X mental retardation gene 1 (FMR1) gene and Fragile X Syndrome.
The C9orf72 gene codes for the protein C9orf72 which is found throughout the brain in neuronal cytoplasm and at presynaptic terminals. Mutations of the C9orf72 gene have been linked to the development of FTD and ALS. These two diseases have a causal relationship to GGGGCC (G4C2) repeats within the 1st intron of C9orf72 gene. Normal individuals typically have around 2 to 8 G4C2 repeats, but individuals with FTD or ALS have from 500 to several thousand G4C2 repeats. The transcribed RNA of these repeats have been shown to form stable G-quadruplexes, with evidence showing that the G4C2 repeats in DNA have the ability to form mixed parallel-antiparallel G-quadruplex structures as well. These RNA transcripts containing G4C2 repeats were shown to bind and separate a wide variety of proteins, including nucleolin. Nucleolin is involved in the synthesis and maturation of ribosomes within the nucleus, and separation of nucleolin by the mutated RNA transcripts impairs nucleolar function and ribosomal RNA synthesis.
Fragile X mental retardation protein (FMRP) is a widely expressed protein coded by the FMR1 gene that binds to G-quadruplex secondary structures in neurons and is involved in synaptic plasticity. FMRP acts as a negative regulator of translation, and its binding stabilizes G-quadruplex structures in mRNA transcripts, inhibiting ribosome elongation of mRNA in the neuron's dendrite and controlling the timing of the transcript's expression. Mutations of this gene can cause the development of Fragile X Syndrome, autism, and other neurological disorders. Specifically, Fragile X Syndrome is caused by an increase from 50 to over 200 CGG repeats within exon 13 of the FMR1 gene. This repeat expansion promotes DNA methylation and other epigenetic heterochromatin modifications of FMR1 that prevent the transcription of the gene, leading to pathological low levels of FMRP.
Therapeutic approaches
Antisense-mediated interventions and small-molecule ligands are common strategies used to target neurological diseases linked to G-quadruplex expansion repeats. Therefore, these techniques are especially advantageous for targeting neurological diseases that have a gain-of-function mechanism, which is when the altered gene product has a new function or new expression of a gene; this has been detected in the C9orf72 (chromosome 9 open reading frame 72).
Antisense therapy is the process by which synthesized strands of nucleic acids are used to bind directly and specifically to the mRNA produced by a certain gene, which will inactivate it. Antisense oligonucleotides (ASOs) are commonly used to target C9orf72 RNA of the G-quadruplex GGGGCC expansion repeat region, which has lowered the toxicity in cellular models of C9orf72. ASOs have previously been used to restore normal phenotypes in other neurological diseases that have gain-of-function mechanisms, the only difference is that it was used in the absence of G-quadruplex expansion repeat regions.
The G-quadruplex decoy strategy is another promising approach for targeting cancer cells by exploiting the unique structural features of the G-quadruplex. The strategy involves designing synthetic oligonucleotides that mimic the G-quadruplex structure and compete with the endogenous G-quadruplexes for binding to transcription factors. These decoys are typically composed of a G-rich sequence that can form a stable G-quadruplex structure and a short linker region that can be modified to optimize their properties. When introduced to cancer cells the decoy can intercept associated transcription factors and bind them leading to the regulation of gene expression. Decoys have been successfully demonstrated to inhibit oncogenic KRAS in SCID mice leading to reduced tumour growth and increased median survival time.
Another commonly used technique is the utilization of small-molecule ligands. These can be used to target G-quadruplex regions that cause neurological disorders. Approximately 1,000 various G-quadruplex ligands exist in which they are able to interact via their aromatic rings; this allows the small-molecule ligands to stack on the planar terminal tetrads within the G-quadruplex regions. A disadvantage of using small-molecule ligands as a therapeutic technique is that specificity is difficult to manage due to the variability of G-quadruplexes in their primary sequences, orientation, thermodynamic stability, and nucleic acid strand stoichiometry. As of now, no single small-molecule ligand has been able to be perfectly specific for a single G-quadruplex sequence. However, a cationic porphyrin known as TMPyP4 is able to bind to the C9orf72 GGGGCC repeat region, which causes the G-quadruplex repeat region to unfold and lose its interactions with proteins causing it to lose its functionality. Small-molecule ligands, composed primarily of lead, can target GGGGCC repeat regions as well and ultimately decreased both repeat-associated non-ATG translation and RNA foci in neuron cells derived from patients with Amyotrophic lateral sclerosis (ALS). This provides evidence that small-molecule ligands are an effective and efficient process to target GGGGCC regions, and that specificity for small-molecule ligand binding is a feasible goal for the scientific community.
Metal complexes have a number of features that make them particularly suitable as G4 DNA binders and therefore as potential drugs. While the metal plays largely a structural role in most G4 binders, there are also examples where it interacts directly with G4s by electrostatic interactions or direct coordination with nucleobases.
References
- Capra JA, Paeschke K, Singh M, Zakian VA (July 2010). "G-quadruplex DNA sequences are evolutionarily conserved and associated with distinct genomic features in Saccharomyces cerevisiae". PLOS Computational Biology. 6 (7): e1000861. Bibcode:2010PLSCB...6E0861C. doi:10.1371/journal.pcbi.1000861. PMC 2908698. PMID 20676380.
- Routh ED, Creacy SD, Beerbower PE, Akman SA, Vaughn JP, Smaldino PJ (March 2017). "A G-quadruplex DNA-affinity Approach for Purification of Enzymaticacvly Active G4 Resolvase1". Journal of Visualized Experiments. 121 (121). doi:10.3791/55496. PMC 5409278. PMID 28362374.
- ^ Largy E, Mergny J, Gabelica V (2016). "Chapter 7. Role of Alkali Metal Ions in G-Quadruplex Nucleic Acid Structure and Stability". In Astrid S, Helmut S, Roland KO (eds.). The Alkali Metal Ions: Their Role in Life (PDF). Metal Ions in Life Sciences. Vol. 16. Springer. pp. 203–258. doi:10.1007/978-3-319-21756-7_7. ISBN 978-3-319-21755-0. PMID 26860303.
- ^ Sundquist WI, Klug A (December 1989). "Telomeric DNA dimerizes by formation of guanine tetrads between hairpin loops". Nature. 342 (6251): 825–9. Bibcode:1989Natur.342..825S. doi:10.1038/342825a0. PMID 2601741. S2CID 4357161.
- ^ Sen D, Gilbert W (July 1988). "Formation of parallel four-stranded complexes by guanine-rich motifs in DNA and its implications for meiosis". Nature. 334 (6180): 364–6. Bibcode:1988Natur.334..364S. doi:10.1038/334364a0. PMID 3393228. S2CID 4351855.
- ^ Rawal P, Kummarasetti VB, Ravindran R, Kumar N, Halder K, Sharma R, Mukerji M, Das SK, Chowdhury S (2006). "Genome-wide Prediction of G4 DNA as Regulatory Motifs: Role in Escherichia Coli Global Regulation". Genome Research. 16 (5): 644–655. doi:10.1101/gr.4508806. PMC 1457047. PMID 16651665.
- ^ Borman S (May 28, 2007). "Ascent of quadruplexes nucleic acid structures become promising drug targets". Chemical and Engineering News. 85 (22): 12–17. doi:10.1021/cen-v085n009.p012a.
- Verma A, Halder K, Halder R, Yadav VK, Rawal P, Thakur RK, Mohd F, Sharma A, Chowdhury S (2008). "Genome-wide Computational and Expression Analyses Reveal G-quadruplex DNA Motifs as Conserved Cis-Regulatory Elements in Human and Related Species". Journal of Medicinal Chemistry. 51 (18): 5641–5649. doi:10.1021/jm800448a. PMID 18767830.
- Han H, Hurley LH (April 2000). "G-quadruplex DNA: a potential target for anti-cancer drug design". Trends in Pharmacological Sciences. 21 (4): 136–42. doi:10.1016/s0165-6147(00)01457-7. PMID 10740289.
- Bochman ML, Paeschke K, Zakian VA (November 2012). "DNA secondary structures: stability and function of G-quadruplex structures". Nature Reviews. Genetics. 13 (11): 770–80. doi:10.1038/nrg3296. PMC 3725559. PMID 23032257.
- Yadav VK, Abraham JK, Mani P, Kulshrestha R, Chowdhury S (2008). "QuadBase: Genome-Wide Database of G4 DNA--occurrence and Conservation in Human, Chimpanzee, Mouse and Rat Promoters and 146 Microbes". Nucleic Acids Research. 36 (Database): D381 – D385. doi:10.1093/nar/gkm781. PMC 2238983. PMID 17962308.
- Dhapola P, Chowdhury S (July 2016). "QuadBase2: Web Server for Multiplexed Guanine Quadruplex Mining and Visualization". Nucleic Acids Research. 44 (W1): W277 – W283. doi:10.1093/nar/gkw425. PMC 4987949. PMID 27185890.
- Rhodes D, Lipps HJ (October 2015). "G-quadruplexes and their regulatory roles in biology". Nucleic Acids Research. 43 (18): 8627–37. doi:10.1093/nar/gkv862. PMC 4605312. PMID 26350216.
- Borman S (November 2009). "Promoter quadruplexes folded DNA structures in gene-activation sites may be useful cancer drug targets". Chemical and Engineering News. 87 (44): 28–30. doi:10.1021/cen-v087n044.p028.
- Gellert M, Lipsett MN, Davies DR (December 1962). "Helix formation by guanylic acid". Proceedings of the National Academy of Sciences of the United States of America. 48 (12): 2013–8. Bibcode:1962PNAS...48.2013G. doi:10.1073/pnas.48.12.2013. PMC 221115. PMID 13947099.
- Henderson E, Hardin CC, Walk SK, Tinoco I, Blackburn EH (December 1987). "Telomeric DNA oligonucleotides form novel intramolecular structures containing guanine-guanine base pairs". Cell. 51 (6): 899–908. doi:10.1016/0092-8674(87)90577-0. PMID 3690664. S2CID 37343642.
- Müller, Sebastian; Kumari, Sunita; Rodriguez, Raphaël; Balasubramanian, Shankar (10 October 2010). "Small-molecule-mediated G-quadruplex isolation from human cells". Nature Chemistry. 2 (12): 1095–1098. Bibcode:2010NatCh...2.1095M. doi:10.1038/nchem.842. PMC 3119466. PMID 21107376.
- Rodriguez, Raphaël; Miller, Kyle M; Forment, Josep V; Bradshaw, Charles R; Nikan, Mehran; Britton, Sébastien; Oelschlaegel, Tobias; Xhemalce, Blerta; Balasubramanian, Shankar; Jackson, Stephen P (5 February 2012). "Small-molecule–induced DNA damage identifies alternative DNA structures in human genes". Nature Chemical Biology. 8 (3): 301–310. doi:10.1038/nchembio.780. PMC 3433707. PMID 22306580.
- Simonsson T (April 2001). "G-quadruplex DNA structures--variations on a theme". Biological Chemistry. 382 (4): 621–8. doi:10.1515/BC.2001.073. PMID 11405224. S2CID 43536134.
- Burge S, Parkinson GN, Hazel P, Todd AK, Neidle S (2006). "Quadruplex DNA: sequence, topology and structure". Nucleic Acids Research. 34 (19): 5402–15. doi:10.1093/nar/gkl655. PMC 1636468. PMID 17012276.
- Cao K, Ryvkin P, Johnson FB (May 2012). "Computational detection and analysis of sequences with duplex-derived interstrand G-quadruplex forming potential". Methods. 57 (1): 3–10. doi:10.1016/j.ymeth.2012.05.002. PMC 3701776. PMID 22652626.
- Kudlicki AS (2016). "G-Quadruplexes Involving Both Strands of Genomic DNA Are Highly Abundant and Colocalize with Functional Sites in the Human Genome". PLOS ONE. 11 (1): e0146174. Bibcode:2016PLoSO..1146174K. doi:10.1371/journal.pone.0146174. PMC 4699641. PMID 26727593.
- Murat P, Balasubramanian S (April 2014). "Existence and consequences of G-quadruplex structures in DNA". Current Opinion in Genetics & Development. 25 (25): 22–9. doi:10.1016/j.gde.2013.10.012. PMID 24584093.
- Miyoshi D, Karimata H, Sugimoto N (June 2006). "Hydration regulates thermodynamics of G-quadruplex formation under molecular crowding conditions". Journal of the American Chemical Society. 128 (24): 7957–63. doi:10.1021/ja061267m. PMID 16771510.
- Zheng KW, Chen Z, Hao YH, Tan Z (January 2010). "Molecular crowding creates an essential environment for the formation of stable G-quadruplexes in long double-stranded DNA". Nucleic Acids Research. 38 (1): 327–38. doi:10.1093/nar/gkp898. PMC 2800236. PMID 19858105.
- Endoh T, Rode AB, Takahashi S, Kataoka Y, Kuwahara M, Sugimoto N (February 2016). "Real-Time Monitoring of G-Quadruplex Formation during Transcription". Analytical Chemistry. 88 (4): 1984–9. doi:10.1021/acs.analchem.5b04396. PMID 26810457.
- Wang Q, Liu JQ, Chen Z, Zheng KW, Chen CY, Hao YH, Tan Z (August 2011). "G-quadruplex formation at the 3' end of telomere DNA inhibits its extension by telomerase, polymerase and unwinding by helicase". Nucleic Acids Research. 39 (14): 6229–37. doi:10.1093/nar/gkr164. PMC 3152327. PMID 21441540.
- Schaffitzel C, Berger I, Postberg J, Hanes J, Lipps HJ, Plückthun A (July 2001). "In vitro generated antibodies specific for telomeric guanine-quadruplex DNA react with Stylonychia lemnae macronuclei". Proceedings of the National Academy of Sciences of the United States of America. 98 (15): 8572–7. Bibcode:2001PNAS...98.8572S. doi:10.1073/pnas.141229498. PMC 37477. PMID 11438689.
- Paeschke K, Simonsson T, Postberg J, Rhodes D, Lipps HJ (October 2005). "Telomere end-binding proteins control the formation of G-quadruplex DNA structures in vivo". Nature Structural & Molecular Biology. 12 (10): 847–54. doi:10.1038/nsmb982. PMID 16142245. S2CID 6079323.
- Kar A, Jones N, Arat NÖ, Fishel R, Griffith JD (June 2018). "Long repeating (TTAGGG) n single-stranded DNA self-condenses into compact beaded filaments stabilized by G-quadruplex formation". The Journal of Biological Chemistry. 293 (24): 9473–9485. doi:10.1074/jbc.RA118.002158. PMC 6005428. PMID 29674319.
- Volná A, Bartas M, Karlický V, Nezval J, Kundrátová K, Pečinka P, et al. (July 2021). "G-Quadruplex in Gene Encoding Large Subunit of Plant RNA Polymerase II: A Billion-Year-Old Story". International Journal of Molecular Sciences. 22 (14): 7381. doi:10.3390/ijms22147381. PMC 8306923. PMID 34299001.
- Simonsson T, Pecinka P, Kubista M (March 1998). "DNA tetraplex formation in the control region of c-myc". Nucleic Acids Research. 26 (5): 1167–72. doi:10.1093/nar/26.5.1167. PMC 147388. PMID 9469822.
- Siddiqui-Jain A, Grand CL, Bearss DJ, Hurley LH (September 2002). "Direct evidence for a G-quadruplex in a promoter region and its targeting with a small molecule to repress c-MYC transcription". Proceedings of the National Academy of Sciences of the United States of America. 99 (18): 11593–8. Bibcode:2002PNAS...9911593S. doi:10.1073/pnas.182256799. PMC 129314. PMID 12195017.
- Huppert JL, Balasubramanian S (14 December 2006). "G-quadruplexes in promoters throughout the human genome". Nucleic Acids Research. 35 (2): 406–13. doi:10.1093/nar/gkl1057. PMC 1802602. PMID 17169996.
- Dai J, Dexheimer TS, Chen D, Carver M, Ambrus A, Jones RA, Yang D (February 2006). "An intramolecular G-quadruplex structure with mixed parallel/antiparallel G-strands formed in the human BCL-2 promoter region in solution". Journal of the American Chemical Society. 128 (4): 1096–8. doi:10.1021/ja055636a. PMC 2556172. PMID 16433524.
- ^ Fernando H, Reszka AP, Huppert J, Ladame S, Rankin S, Venkitaraman AR, Neidle S, Balasubramanian S (June 2006). "A conserved quadruplex motif located in a transcription activation site of the human c-kit oncogene". Biochemistry. 45 (25): 7854–60. doi:10.1021/bi0601510. PMC 2195898. PMID 16784237.
- Huppert JL, Balasubramanian S (2005). "Prevalence of quadruplexes in the human genome". Nucleic Acids Research. 33 (9): 2908–16. doi:10.1093/nar/gki609. PMC 1140081. PMID 15914667.
- Rawal P, Kummarasetti VB, Ravindran J, Kumar N, Halder K, Sharma R, Mukerji M, Das SK, Chowdhury S (May 2006). "Genome-wide prediction of G4 DNA as regulatory motifs: role in Escherichia coli global regulation". Genome Research. 16 (5): 644–55. doi:10.1101/gr.4508806. PMC 1457047. PMID 16651665.
- Kamath-Loeb A, Loeb LA, Fry M (2012). Cotterill S (ed.). "The Werner syndrome protein is distinguished from the Bloom syndrome protein by its capacity to tightly bind diverse DNA structures". PLOS ONE. 7 (1): e30189. Bibcode:2012PLoSO...730189K. doi:10.1371/journal.pone.0030189. PMC 3260238. PMID 22272300.
- Vaughn JP, Creacy SD, Routh ED, Joyner-Butt C, Jenkins GS, Pauli S, Nagamine Y, Akman SA (November 2005). "The DEXH protein product of the DHX36 gene is the major source of tetramolecular quadruplex G4-DNA resolving activity in HeLa cell lysates". The Journal of Biological Chemistry. 280 (46): 38117–20. doi:10.1074/jbc.C500348200. PMID 16150737.
- Chen MC, Ferré-D'Amaré AR (15 August 2017). "Structural Basis of DEAH/RHA Helicase Activity". Crystals. 7 (8): 253. doi:10.3390/cryst7080253.
- ^ Thakur RK, Kumar P, Halder K, Verma A, Kar A, Parent JL, Basundra R, Kumar A, Chowdhury S (January 2009). "Metastases Suppressor NM23-H2 Interaction With G-quadruplex DNA Within c-MYC Promoter Nuclease Hypersensitive Element Induces c-MYC Expression". Nucleic Acids Research. 37 (1): 172–183. doi:10.1093/nar/gkn919. PMC 2615625. PMID 19033359.
- Borman S (November 2009). "Promoter Quadruplexes Folded DNA structures in gene-activation sites may be useful cancer drug targets". Chemical and Engineering News. 87 (44): 28–30. doi:10.1021/cen-v087n044.p028.
- ^ Saha D, Singh A, Hussain T, Srivastava V, Sengupta S, Kar A, Dhapola P, Ummanni R, Chowdhury S (July 2017). "Epigenetic Suppression of Human Telomerase ( hTERT) Is Mediated by the Metastasis Suppressor NME2 in a G-quadruplex-dependent Fashion". The Journal of Biological Chemistry. 292 (37): 15205–15215. doi:10.1074/jbc.M117.792077. PMC 5602382. PMID 28717007.
- Mukherjee AK, Sharma S, Bagri S, Kutum R, Kumar P, Hussain A, Singh P, Saha D, Kar A, Dash D, Chowdhury S (November 2019). "Telomere Repeat-Binding Factor 2 Binds Extensively to Extra-Telomeric G-quadruplexes and Regulates the Epigenetic Status of Several Gene Promoters". The Journal of Biological Chemistry. 294 (47): 17709–17722. doi:10.1074/jbc.RA119.008687. PMC 6879327. PMID 31575660.
- Maizels N, Gray LT (April 2013). Rosenberg SM (ed.). "The G4 genome". PLOS Genetics. 9 (4): e1003468. doi:10.1371/journal.pgen.1003468. PMC 3630100. PMID 23637633.
- Biffi G, Tannahill D, McCafferty J, Balasubramanian S (March 2013). "Quantitative visualization of DNA G-quadruplex structures in human cells". Nature Chemistry. 5 (3): 182–6. Bibcode:2013NatCh...5..182B. doi:10.1038/nchem.1548. PMC 3622242. PMID 23422559.
- Chen MC, Tippana R, Demeshkina NA, Murat P, Balasubramanian S, Myong S, Ferré-D'Amaré AR (June 2018). "Structural basis of G-quadruplex unfolding by the DEAH/RHA helicase DHX36". Nature. 558 (7710): 465–469. Bibcode:2018Natur.558..465C. doi:10.1038/s41586-018-0209-9. PMC 6261253. PMID 29899445.
- Rice C, Skordalakes E (2016). "Structure and function of the telomeric CST complex". Computational and Structural Biotechnology Journal. 14: 161–7. doi:10.1016/j.csbj.2016.04.002. PMC 4872678. PMID 27239262.
- Hänsel-Hertsch R, Beraldi D, Lensing SV, Marsico G, Zyner K, Parry A, et al. (October 2016). "G-quadruplex structures mark human regulatory chromatin". Nature Genetics. 48 (10): 1267–1272. doi:10.1038/ng.3662. PMID 27618450. S2CID 20967177.
- ^ Poetsch AR (2020). "AP-Seq: A Method to Measure Apurinic Sites and Small Base Adducts Genome-Wide". The Nucleus. Methods in Molecular Biology. Vol. 2175. Clifton, N.J. pp. 95–108. doi:10.1007/978-1-0716-0763-3_8. ISBN 978-1-0716-0762-6. ISSN 1940-6029. PMID 32681486. S2CID 220631202.
{{cite book}}
: CS1 maint: location missing publisher (link) - ^ Roychoudhury S, Pramanik S, Harris HL, Tarpley M, Sarkar A, Spagnol G, et al. (May 2020). "Endogenous oxidized DNA bases and APE1 regulate the formation of G-quadruplex structures in the genome". Proceedings of the National Academy of Sciences of the United States of America. 117 (21): 11409–11420. Bibcode:2020PNAS..11711409R. doi:10.1073/pnas.1912355117. PMC 7260947. PMID 32404420.
- ^ Canugovi C, Shamanna RA, Croteau DL, Bohr VA (June 2014). "Base excision DNA repair levels in mitochondrial lysates of Alzheimer's disease". Neurobiology of Aging. 35 (6): 1293–1300. doi:10.1016/j.neurobiolaging.2014.01.004. PMC 5576885. PMID 24485507.
- ^ Sun D, Hurley LH (May 2009). "The importance of negative superhelicity in inducing the formation of G-quadruplex and i-motif structures in the c-Myc promoter: implications for drug targeting and control of gene expression". Journal of Medicinal Chemistry. 52 (9): 2863–2874. doi:10.1021/jm900055s. PMC 2757002. PMID 19385599.
- ^ Hill JW, Hazra TK, Izumi T, Mitra S (January 2001). "Stimulation of human 8-oxoguanine-DNA glycosylase by AP-endonuclease: potential coordination of the initial steps in base excision repair". Nucleic Acids Research. 29 (2): 430–438. doi:10.1093/nar/29.2.430. PMC 29662. PMID 11139613.
- Burrows CJ, Muller JG (May 1998). "Oxidative Nucleobase Modifications Leading to Strand Scission". Chemical Reviews. 98 (3): 1109–1152. doi:10.1021/cr960421s. PMID 11848927.
- ^ Poetsch AR (2020-01-07). "The genomics of oxidative DNA damage, repair, and resulting mutagenesis". Computational and Structural Biotechnology Journal. 18: 207–219. doi:10.1016/j.csbj.2019.12.013. PMC 6974700. PMID 31993111.
- ^ Fleming AM, Burrows CJ (October 2017). "8-Oxo-7,8-dihydro-2'-deoxyguanosine and abasic site tandem lesions are oxidation prone yielding hydantoin products that strongly destabilize duplex DNA". Organic & Biomolecular Chemistry. 15 (39): 8341–8353. doi:10.1039/C7OB02096A. PMC 5636683. PMID 28936535.
- ^ Kitsera N, Rodriguez-Alvarez M, Emmert S, Carell T, Khobta A (September 2019). "Nucleotide excision repair of abasic DNA lesions". Nucleic Acids Research. 47 (16): 8537–8547. doi:10.1093/nar/gkz558. PMC 6895268. PMID 31226203.
- ^ Roychoudhury S, Nath S, Song H, Hegde ML, Bellot LJ, Mantha AK, et al. (March 2017). "Human Apurinic/Apyrimidinic Endonuclease (APE1) Is Acetylated at DNA Damage Sites in Chromatin, and Acetylation Modulates Its DNA Repair Activity". Molecular and Cellular Biology. 37 (6). doi:10.1128/mcb.00401-16. PMC 5335514. PMID 27994014.
- Chattopadhyay R, Das S, Maiti AK, Boldogh I, Xie J, Hazra TK, et al. (December 2008). "Regulatory role of human AP-endonuclease (APE1/Ref-1) in YB-1-mediated activation of the multidrug resistance gene MDR1". Molecular and Cellular Biology. 28 (23): 7066–7080. doi:10.1128/mcb.00244-08. PMC 2593380. PMID 18809583.
- Bhakat KK, Izumi T, Yang SH, Hazra TK, Mitra S (December 2003). "Role of acetylated human AP-endonuclease (APE1/Ref-1) in regulation of the parathyroid hormone gene". The EMBO Journal. 22 (23): 6299–6309. doi:10.1093/emboj/cdg595. PMC 291836. PMID 14633989.
- Yamamori T, DeRicco J, Naqvi A, Hoffman TA, Mattagajasingh I, Kasuno K, et al. (January 2010). "SIRT1 deacetylates APE1 and regulates cellular base excision repair". Nucleic Acids Research. 38 (3): 832–845. doi:10.1093/nar/gkp1039. PMC 2817463. PMID 19934257.
- Neidle S (July 2016). "Quadruplex Nucleic Acids as Novel Therapeutic Targets" (PDF). Journal of Medicinal Chemistry. 59 (13): 5987–6011. doi:10.1021/acs.jmedchem.5b01835. PMID 26840940.
- Chen Y, Yang D (September 2012). Sequence, stability, and structure of G-quadruplexes and their interactions with drugs. Vol. Chapter 17. pp. 17.5.1–17.5.17. doi:10.1002/0471142700.nc1705s50. ISBN 978-0471142706. PMC 3463244. PMID 22956454.
{{cite book}}
:|journal=
ignored (help) - Brooks TA, Kendrick S, Hurley L (September 2010). "Making sense of G-quadruplex and i-motif functions in oncogene promoters". The FEBS Journal. 277 (17): 3459–69. doi:10.1111/j.1742-4658.2010.07759.x. PMC 2971675. PMID 20670278.
- ^ Ou TM, Lin J, Lu YJ, Hou JQ, Tan JH, Chen SH, Li Z, Li YP, Li D, Gu LQ, Huang ZS (August 2011). "Inhibition of cell proliferation by quindoline derivative (SYUIQ-05) through its preferential interaction with c-myc promoter G-quadruplex". Journal of Medicinal Chemistry. 54 (16): 5671–9. doi:10.1021/jm200062u. PMID 21774525.
- Sharma S, Mukherjee AK, Roy SS, Bagri S, Lier S, Verma M, Sengupta A, Kumar M, Nesse G, Pandey DP, Chowdhury S (January 2020). "Human Telomerase Expression is under Direct Transcriptional Control of the Telomere-binding-factor TRF2" (PDF). bioRxiv. doi:10.1101/2020.01.15.907626. S2CID 214472968.
- Sun D, Guo K, Rusche JJ, Hurley LH (2005-10-12). "Facilitation of a structural transition in the polypurine/polypyrimidine tract within the proximal promoter region of the human VEGF gene by the presence of potassium and G-quadruplex-interactive agents". Nucleic Acids Research. 33 (18): 6070–80. doi:10.1093/nar/gki917. PMC 1266068. PMID 16239639.
- Hussain T, Saha D, Purohit G, Mukherjee AK, Sharma S, Sengupta S, Dhapola P, Maji B, Vedagopuram S, Horikoshi NT, Horikoshi N, Pandita RK, Bhattacharya S, Bajaj A, Riou JF, Pandita TK, Chowdhury S (September 2017). "Transcription Regulation of CDKN1A (p21/CIP1/WAF1) by TRF2 Is Epigenetically Controlled Through the REST Repressor Complex". Scientific Reports. 7 (1): 11541. Bibcode:2017NatSR...711541H. doi:10.1038/s41598-017-11177-1. PMC 5599563. PMID 28912501.
- De Armond R, Wood S, Sun D, Hurley LH, Ebbinghaus SW (December 2005). "Evidence for the presence of a guanine quadruplex forming region within a polypurine tract of the hypoxia inducible factor 1alpha promoter". Biochemistry. 44 (49): 16341–50. doi:10.1021/bi051618u. PMID 16331995.
- Guo K, Pourpak A, Beetz-Rogers K, Gokhale V, Sun D, Hurley LH (August 2007). "Formation of pseudosymmetrical G-quadruplex and i-motif structures in the proximal promoter region of the RET oncogene". Journal of the American Chemical Society. 129 (33): 10220–8. doi:10.1021/ja072185g. PMC 2566970. PMID 17672459.
- Qin Y, Rezler EM, Gokhale V, Sun D, Hurley LH (2007-11-26). "Characterization of the G-quadruplexes in the duplex nuclease hypersensitive element of the PDGF-A promoter and modulation of PDGF-A promoter activity by TMPyP4". Nucleic Acids Research. 35 (22): 7698–713. doi:10.1093/nar/gkm538. PMC 2190695. PMID 17984069.
- Chilakamarthi U, Koteshwar D, Jinka S, Vamsi Krishna N, Sridharan K, Nagesh N, Giribabu L (November 2018). "Novel Amphiphilic G-Quadruplex Binding Synthetic Derivative of TMPyP4 and Its Effect on Cancer Cell Proliferation and Apoptosis Induction". Biochemistry. 57 (46): 6514–6527. doi:10.1021/acs.biochem.8b00843. PMID 30369235. S2CID 53093959.
- Ohnmacht SA, Marchetti C, Gunaratnam M, Besser RJ, Haider SM, Di Vita G, Lowe HL, Mellinas-Gomez M, Diocou S, Robson M, Šponer J, Islam B, Pedley RB, Hartley JA, Neidle S (June 2015). "A G-quadruplex-binding compound showing anti-tumour activity in an in vivo model for pancreatic cancer". Scientific Reports. 5: 11385. Bibcode:2015NatSR...511385O. doi:10.1038/srep11385. PMC 4468576. PMID 26077929.
- Siddiqui-Jain A, Grand CL, Bearss DJ, Hurley LH (September 2002). "Direct evidence for a G-quadruplex in a promoter region and its targeting with a small molecule to repress c-MYC transcription". Proceedings of the National Academy of Sciences of the United States of America. 99 (18): 11593–8. Bibcode:2002PNAS...9911593S. doi:10.1073/pnas.182256799. PMC 129314. PMID 12195017.
- Collie GW, Promontorio R, Hampel SM, Micco M, Neidle S, Parkinson GN (February 2012). "Structural basis for telomeric G-quadruplex targeting by naphthalene diimide ligands". Journal of the American Chemical Society. 134 (5): 2723–31. doi:10.1021/ja2102423. PMID 22280460.
- Todd AK, Johnston M, Neidle S (2005). "Highly prevalent putative quadruplex sequence motifs in human DNA". Nucleic Acids Research. 33 (9): 2901–7. doi:10.1093/nar/gki553. PMC 1140077. PMID 15914666.
- Frank-Kamenetskii MD, Mirkin SM (1995). "Triplex DNA structures". Annual Review of Biochemistry. 64 (9): 65–95. doi:10.1146/annurev.bi.64.070195.000433. PMID 7574496.
- Guo K, Gokhale V, Hurley LH, Sun D (August 2008). "Intramolecularly folded G-quadruplex and i-motif structures in the proximal promoter of the vascular endothelial growth factor gene". Nucleic Acids Research. 36 (14): 4598–608. doi:10.1093/nar/gkn380. PMC 2504309. PMID 18614607.
- Mirkin SM, Lyamichev VI, Drushlyak KN, Dobrynin VN, Filippov SA, Frank-Kamenetskii MD (1987). "DNA H form requires a homopurine-homopyrimidine mirror repeat". Nature. 330 (6147): 495–7. Bibcode:1987Natur.330..495M. doi:10.1038/330495a0. PMID 2825028. S2CID 4360764.
- Smith SS (2010). "Evolutionary expansion of structurally complex DNA sequences". Cancer Genomics & Proteomics. 7 (4): 207–15. PMID 20656986.
- Brázda, Václav; Kolomazník, Jan; Lýsek, Jiří; Bartas, Martin; Fojta, Miroslav; Šťastný, Jiří; Mergny, Jean-Louis (2019-09-15). Hancock, John (ed.). "G4Hunter web application: a web server for G-quadruplex prediction". Bioinformatics. 35 (18): 3493–3495. doi:10.1093/bioinformatics/btz087. ISSN 1367-4803. PMC 6748775. PMID 30721922.
- Garant, Jean-Michel; Perreault, Jean-Pierre; Scott, Michelle S. (2018). "G4RNA screener web server: User focused interface for RNA G-quadruplex prediction". Biochimie. 151: 115–118. doi:10.1016/j.biochi.2018.06.002. PMID 29885355. S2CID 47005625.
- Kwok CK, Merrick CJ (October 2017). "G-Quadruplexes: Prediction, Characterization, and Biological Application" (PDF). Trends in Biotechnology. 35 (10): 997–1013. doi:10.1016/j.tibtech.2017.06.012. PMID 28755976.
- Han H, Hurley LH, Salazar M (January 1999). "A DNA polymerase stop assay for G-quadruplex-interactive compounds". Nucleic Acids Research. 27 (2): 537–542. doi:10.1093/nar/27.2.537. PMC 148212. PMID 9862977.
- Sun D, Hurley LH (2009-10-23). "Biochemical Techniques for the Characterization of G-Quadruplex Structures: EMSA, DMS Footprinting, and DNA Polymerase Stop Assay". G-Quadruplex DNA. Methods in Molecular Biology. Vol. 608. Humana Press. pp. 65–79. doi:10.1007/978-1-59745-363-9_5. ISBN 9781588299505. PMC 2797547. PMID 20012416.
- Paramasivan S, Rujan I, Bolton PH (December 2007). "Circular dichroism of quadruplex DNAs: applications to structure, cation effects and ligand binding". Methods. 43 (4): 324–331. doi:10.1016/j.ymeth.2007.02.009. PMID 17967702.
- Mergny JL, Phan AT, Lacroix L (September 1998). "Following G-quartet formation by UV-spectroscopy". FEBS Letters. 435 (1): 74–78. Bibcode:1998FEBSL.435...74M. doi:10.1016/s0014-5793(98)01043-6. PMID 9755862. S2CID 1306129.
- Dao, N.T. (2011). "Following G-quadruplex formation by its intrinsic fluorescence". FEBS Letters. 585 (24): 3969–3977. Bibcode:2011FEBSL.585.3969D. doi:10.1016/j.febslet.2011.11.004. hdl:10356/98618. PMID 22079665.
- Markovitsi, D. (2004). "Cooperative effects in the photophysical properties of self-associated triguanosine diphosphates". Photochem. Photobiol. 79 (6): 526–530. doi:10.1562/2003-12-12-RA.1.
- An N, Fleming AM, Middleton EG, Burrows CJ (October 2014). "Single-molecule investigation of G-quadruplex folds of the human telomere sequence in a protein nanocavity". Proceedings of the National Academy of Sciences of the United States of America. 111 (40): 14325–14331. Bibcode:2014PNAS..11114325A. doi:10.1073/pnas.1415944111. PMC 4209999. PMID 25225404.
- Bošković F, Zhu J, Chen K, Keyser UF (November 2019). "Monitoring G-Quadruplex Formation with DNA Carriers and Solid-State Nanopores". Nano Letters. 19 (11): 7996–8001. Bibcode:2019NanoL..19.7996B. doi:10.1021/acs.nanolett.9b03184. PMID 31577148. S2CID 203638480.
- Simone R, Fratta P, Neidle S, Parkinson GN, Isaacs AM (June 2015). "G-quadruplexes: Emerging roles in neurodegenerative diseases and the non-coding transcriptome". FEBS Letters. 589 (14): 1653–68. Bibcode:2015FEBSL.589.1653S. doi:10.1016/j.febslet.2015.05.003. PMID 25979174.
- "C9orf72 chromosome 9 open reading frame 72 [Homo sapiens] - Gene]". National Center for Biotechnology Information. U.S. National Library of Medicine.
- Ratnavalli E, Brayne C, Dawson K, Hodges JR (June 2002). "The prevalence of frontotemporal dementia". Neurology. 58 (11): 1615–21. doi:10.1212/WNL.58.11.1615. PMID 12058088. S2CID 45904851.
- Rutherford NJ, Heckman MG, Dejesus-Hernandez M, Baker MC, Soto-Ortolaza AI, Rayaprolu S, Stewart H, Finger E, Volkening K, Seeley WW, Hatanpaa KJ, Lomen-Hoerth C, Kertesz A, Bigio EH, Lippa C, Knopman DS, Kretzschmar HA, Neumann M, Caselli RJ, White CL, Mackenzie IR, Petersen RC, Strong MJ, Miller BL, Boeve BF, Uitti RJ, Boylan KB, Wszolek ZK, Graff-Radford NR, Dickson DW, Ross OA, Rademakers R (December 2012). "Length of normal alleles of C9ORF72 GGGGCC repeat do not influence disease phenotype". Neurobiology of Aging. 33 (12): 2950.e5–7. doi:10.1016/j.neurobiolaging.2012.07.005. PMC 3617405. PMID 22840558.
- Beck J, Poulter M, Hensman D, Rohrer JD, Mahoney CJ, Adamson G, Campbell T, Uphill J, Borg A, Fratta P, Orrell RW, Malaspina A, Rowe J, Brown J, Hodges J, Sidle K, Polke JM, Houlden H, Schott JM, Fox NC, Rossor MN, Tabrizi SJ, Isaacs AM, Hardy J, Warren JD, Collinge J, Mead S (March 2013). "Large C9orf72 hexanucleotide repeat expansions are seen in multiple neurodegenerative syndromes and are more frequent than expected in the UK population". American Journal of Human Genetics. 92 (3): 345–53. doi:10.1016/j.ajhg.2013.01.011. PMC 3591848. PMID 23434116.
- Fratta P, Mizielinska S, Nicoll AJ, Zloh M, Fisher EM, Parkinson G, Isaacs AM (December 2012). "C9orf72 hexanucleotide repeat associated with amyotrophic lateral sclerosis and frontotemporal dementia forms RNA G-quadruplexes". Scientific Reports. 2: 1016. Bibcode:2012NatSR...2E1016F. doi:10.1038/srep01016. PMC 3527825. PMID 23264878.
- Reddy K, Zamiri B, Stanley SY, Macgregor RB, Pearson CE (April 2013). "The disease-associated r(GGGGCC)n repeat from the C9orf72 gene forms tract length-dependent uni- and multimolecular RNA G-quadruplex structures". The Journal of Biological Chemistry. 288 (14): 9860–6. doi:10.1074/jbc.C113.452532. PMC 3617286. PMID 23423380.
- Haeusler AR, Donnelly CJ, Periz G, Simko EA, Shaw PG, Kim MS, Maragakis NJ, Troncoso JC, Pandey A, Sattler R, Rothstein JD, Wang J (March 2014). "C9orf72 nucleotide repeat structures initiate molecular cascades of disease". Nature. 507 (7491): 195–200. Bibcode:2014Natur.507..195H. doi:10.1038/nature13124. PMC 4046618. PMID 24598541.
- Darnell JC, Jensen KB, Jin P, Brown V, Warren ST, Darnell RB (November 2001). "Fragile X mental retardation protein targets G quartet mRNAs important for neuronal function". Cell. 107 (4): 489–499. doi:10.1016/S0092-8674(01)00566-9. PMID 11719189. S2CID 8203054.
- Ceman S, O'Donnell WT, Reed M, Patton S, Pohl J, Warren ST (December 2003). "Phosphorylation influences the translation state of FMRP-associated polyribosomes". Human Molecular Genetics. 12 (24): 3295–3305. doi:10.1093/hmg/ddg350. PMID 14570712.
- Fähling M, Mrowka R, Steege A, Kirschner KM, Benko E, Förstera B, et al. (February 2009). "Translational regulation of the human achaete-scute homologue-1 by fragile X mental retardation protein". The Journal of Biological Chemistry. 284 (7): 4255–4266. doi:10.1074/jbc.M807354200. PMID 19097999.
- "Fragile X Mental Retardation". Gene Cards.
- Pieretti M, Zhang FP, Fu YH, Warren ST, Oostra BA, Caskey CT, Nelson DL (August 1991). "Absence of expression of the FMR-1 gene in fragile X syndrome". Cell. 66 (4): 817–822. doi:10.1016/0092-8674(91)90125-I. PMID 1878973. S2CID 31455523.
- Sutcliffe JS, Nelson DL, Zhang F, Pieretti M, Caskey CT, Saxe D, Warren ST (September 1992). "DNA methylation represses FMR-1 transcription in fragile X syndrome". Human Molecular Genetics. 1 (6): 397–400. doi:10.1093/hmg/1.6.397. PMID 1301913.
- Mizielinska S, Isaacs AM (October 2014). "C9orf72 amyotrophic lateral sclerosis and frontotemporal dementia: gain or loss of function?". Current Opinion in Neurology. 27 (5): 515–23. doi:10.1097/WCO.0000000000000130. PMC 4165481. PMID 25188012.
- Donnelly CJ, Zhang PW, Pham JT, Haeusler AR, Heusler AR, Mistry NA, Vidensky S, Daley EL, Poth EM, Hoover B, Fines DM, Maragakis N, Tienari PJ, Petrucelli L, Traynor BJ, Wang J, Rigo F, Bennett CF, Blackshaw S, Sattler R, Rothstein JD (October 2013). "RNA toxicity from the ALS/FTD C9ORF72 expansion is mitigated by antisense intervention". Neuron. 80 (2): 415–28. doi:10.1016/j.neuron.2013.10.015. PMC 4098943. PMID 24139042.
- Lagier-Tourenne C, Baughn M, Rigo F, Sun S, Liu P, Li HR, Jiang J, Watt AT, Chun S, Katz M, Qiu J, Sun Y, Ling SC, Zhu Q, Polymenidou M, Drenner K, Artates JW, McAlonis-Downes M, Markmiller S, Hutt KR, Pizzo DP, Cady J, Harms MB, Baloh RH, Vandenberg SR, Yeo GW, Fu XD, Bennett CF, Cleveland DW, Ravits J (November 2013). "Targeted degradation of sense and antisense C9orf72 RNA foci as therapy for ALS and frontotemporal degeneration". Proceedings of the National Academy of Sciences of the United States of America. 110 (47): E4530–9. Bibcode:2013PNAS..110E4530L. doi:10.1073/pnas.1318835110. PMC 3839752. PMID 24170860.
- Sareen D, O'Rourke JG, Meera P, Muhammad AK, Grant S, Simpkinson M, Bell S, Carmona S, Ornelas L, Sahabian A, Gendron T, Petrucelli L, Baughn M, Ravits J, Harms MB, Rigo F, Bennett CF, Otis TS, Svendsen CN, Baloh RH (October 2013). "Targeting RNA foci in iPSC-derived motor neurons from ALS patients with a C9ORF72 repeat expansion". Science Translational Medicine. 5 (208): 208ra149. doi:10.1126/scitranslmed.3007529. PMC 4090945. PMID 24154603.
- Wheeler TM, Leger AJ, Pandey SK, MacLeod AR, Nakamori M, Cheng SH, Wentworth BM, Bennett CF, Thornton CA (August 2012). "Targeting nuclear RNA for in vivo correction of myotonic dystrophy". Nature. 488 (7409): 111–5. Bibcode:2012Natur.488..111W. doi:10.1038/nature11362. PMC 4221572. PMID 22859208.
- Lee JE, Bennett CF, Cooper TA (March 2012). "RNase H-mediated degradation of toxic RNA in myotonic dystrophy type 1". Proceedings of the National Academy of Sciences of the United States of America. 109 (11): 4221–6. Bibcode:2012PNAS..109.4221L. doi:10.1073/pnas.1117019109. PMC 3306674. PMID 22371589.
- Carroll JB, Warby SC, Southwell AL, Doty CN, Greenlee S, Skotte N, Hung G, Bennett CF, Freier SM, Hayden MR (December 2011). "Potent and selective antisense oligonucleotides targeting single-nucleotide polymorphisms in the Huntington disease gene / allele-specific silencing of mutant huntingtin". Molecular Therapy. 19 (12): 2178–85. doi:10.1038/mt.2011.201. PMC 3242664. PMID 21971427.
- Gagnon KT, Pendergraff HM, Deleavey GF, Swayze EE, Potier P, Randolph J, Roesch EB, Chattopadhyaya J, Damha MJ, Bennett CF, Montaillier C, Lemaitre M, Corey DR (November 2010). "Allele-selective inhibition of mutant huntingtin expression with antisense oligonucleotides targeting the expanded CAG repeat". Biochemistry. 49 (47): 10166–78. doi:10.1021/bi101208k. PMC 2991413. PMID 21028906.
- Cogoi S, Paramasivam M, Filichev V, Géci I, Pedersen EB, Xodo LE (January 2009). "Identification of a new G-quadruplex motif in the KRAS promoter and design of pyrene-modified G4-decoys with antiproliferative activity in pancreatic cancer cells". Journal of Medicinal Chemistry. 52 (2): 564–568. doi:10.1021/jm800874t. PMID 19099510.
- Cogoi S, Zorzet S, Rapozzi V, Géci I, Pedersen EB, Xodo LE (April 2013). "MAZ-binding G4-decoy with locked nucleic acid and twisted intercalating nucleic acid modifications suppresses KRAS in pancreatic cancer cells and delays tumor growth in mice". Nucleic Acids Research. 41 (7): 4049–4064. doi:10.1093/nar/gkt127. PMC 3627599. PMID 23471001.
- Campbell NH, Patel M, Tofa AB, Ghosh R, Parkinson GN, Neidle S (March 2009). "Selectivity in ligand recognition of G-quadruplex loops". Biochemistry. 48 (8): 1675–80. doi:10.1021/bi802233v. PMID 19173611.
- Ohnmacht SA, Neidle S (June 2014). "Small-molecule quadruplex-targeted drug discovery". Bioorganic & Medicinal Chemistry Letters. 24 (12): 2602–12. doi:10.1016/j.bmcl.2014.04.029. PMID 24814531.
- Zamiri B, Reddy K, Macgregor RB, Pearson CE (February 2014). "TMPyP4 porphyrin distorts RNA G-quadruplex structures of the disease-associated r(GGGGCC)n repeat of the C9orf72 gene and blocks interaction of RNA-binding proteins". The Journal of Biological Chemistry. 289 (8): 4653–9. doi:10.1074/jbc.C113.502336. PMC 3931028. PMID 24371143.
- Vilar R (2018). "Chapter 12. Nucleic Acid Quadruplexes and Metallo-Drugs". In Sigel A, Sigel H, Freisinger E, Sigel RK (eds.). Metallo-Drugs: Development and Action of Anticancer Agents. Vol. 18. pp. 325–349. doi:10.1515/9783110470734-018. ISBN 9783110470734. PMID 29394031. S2CID 44981950.
{{cite book}}
:|journal=
ignored (help)
Further reading
- Ren J, Wang J, Han L, Wang E, Wang J (October 2011). "Kinetically grafting G-quadruplexes onto DNA nanostructures for structure and function encoding via a DNA machine". Chemical Communications. 47 (38): 10563–5. doi:10.1039/c1cc13973h. PMID 21858307.
- Johnson JE, Smith JS, Kozak ML, Johnson FB (August 2008). "In vivo veritas: using yeast to probe the biological functions of G-quadruplexes". Biochimie. 90 (8): 1250–63. doi:10.1016/j.biochi.2008.02.013. PMC 2585026. PMID 18331848.
- Huppert JL, Balasubramanian S (2005). "Prevalence of quadruplexes in the human genome". Nucleic Acids Research. 33 (9): 2908–16. doi:10.1093/nar/gki609. PMC 1140081. PMID 15914667.
- Todd AK, Johnston M, Neidle S (2005). "Highly prevalent putative quadruplex sequence motifs in human DNA". Nucleic Acids Research. 33 (9): 2901–7. doi:10.1093/nar/gki553. PMC 1140077. PMID 15914666.
- Burge S, Parkinson GN, Hazel P, Todd AK, Neidle S (2006). "Quadruplex DNA: sequence, topology and structure". Nucleic Acids Research. 34 (19): 5402–15. doi:10.1093/nar/gkl655. PMC 1636468. PMID 17012276.
- Siddiqui-Jain A, Grand CL, Bearss DJ, Hurley LH (September 2002). "Direct evidence for a G-quadruplex in a promoter region and its targeting with a small molecule to repress c-MYC transcription". Proceedings of the National Academy of Sciences of the United States of America. 99 (18): 11593–8. Bibcode:2002PNAS...9911593S. doi:10.1073/pnas.182256799. PMC 129314. PMID 12195017.
- Rawal P, Kummarasetti VB, Ravindran J, Kumar N, Halder K, Sharma R, Mukerji M, Das SK, Chowdhury S (May 2006). "Genome-wide prediction of G4 DNA as regulatory motifs: role in Escherichia coli global regulation". Genome Research. 16 (5): 644–55. doi:10.1101/gr.4508806. PMC 1457047. PMID 16651665.
- Hou X, Guo W, Xia F, Nie FQ, Dong H, Tian Y, Wen L, Wang L, Cao L, Yang Y, Xue J, Song Y, Wang Y, Liu D, Jiang L (June 2009). "A biomimetic potassium responsive nanochannel: G-quadruplex DNA conformational switching in a synthetic nanopore". Journal of the American Chemical Society. 131 (22): 7800–5. doi:10.1021/ja901574c. PMID 19435350.
- Neidle & Balasubramanian, ed. (2006). Quadruplex Nucleic Acids. Royal Society of Chemistry. ISBN 978-0-85404-374-3. Archived from the original on 2007-09-30.
- Rowland GB, Barnett K, Dupont JI, Akurathi G, Le VH, Lewis EA (December 2013). "The effect of pyridyl substituents on the thermodynamics of porphyrin binding to G-quadruplex DNA". Bioorganic & Medicinal Chemistry. 21 (23): 7515–22. doi:10.1016/j.bmc.2013.09.036. PMID 24148836.
External links
Quadruplex websites
- Nanopore and Aptamer Biosensor group{NAB group}
- G-Quadruplex World – a website to discuss publications and other information of interest to those working in the field of G-quadruplexes
- Greglist – a database listing potential G-quadruplex regulated genes
- Database on Quadruplex information: QuadBase from IGIB
- GRSDB- a database of G-quadruplexes near RNA processing sites.
- GRS_UTRdb Archived 2011-07-20 at the Wayback Machine- a database of G-quadruplexes in the UTRs.
- G-quadruplex Resource Site
- non-B Motif Search Tool at non-B DB- a web server to predict G-quadruplex forming motifs and other non-B DNA forming motifs from users' DNA sequences.
Tools to predict G-quadruplex motifs
- QGRS Mapper: a web-based application for predicting G-quadruplexes in nucleotide sequences and NCBI genes from Bagga's group.
- Quadfinder: Tool for Prediction and Analysis of G Quadruplex Motifs in DNA/RNA Sequences from Maiti's group, IGIB, Delhi, India
- G4Hunter from Mergny's group but user need to run the code in R.
- pqsfinder: an exhaustive and imperfection-tolerant search tool for potential quadruplex-forming sequences in R.
- pqsfinder: online search tool using the latest R/Bioconductor package