Revision as of 11:30, 16 October 2010 editSan9663 (talk | contribs)Extended confirmed users564 editsm →Geological distribution← Previous edit | Revision as of 11:58, 16 October 2010 edit undoPhoenix7777 (talk | contribs)Extended confirmed users14,536 edits Reverted edit by San9663 to last revision by Kidela. Per WP:LINK.Next edit → | ||
Line 159: | Line 159: | ||
==Geological distribution== | ==Geological distribution== | ||
The rare earth elements are often found together. However, |
The rare earth elements are often found together. However, Promethium, the only radioactive and hence unstable element, has a very short half life of 20 years and only exists in nature in negligible amounts (approximately 572 g in the earth's crust).<ref>{{cite journal | author = P. Belli, R. Bernabei, F. Cappella, R. Cerulli, C.J. Dai, F.A. Danevich, A. d’Angelo, A. Incicchitti, V.V. Kobychev, S.S. Nagorny, S. Nisi, F. Nozzoli, D. Prosperi, V.I. Tretyak, S.S. Yurchenko | year = 2007 | month = | title = Search for α decay of natural Europium | journal = Nuclear Physics A | volume = 789 | issue = | pages = 15–29 | doi = 10.1016/j.nuclphysa.2007.03.001 }}</ref> | ||
Due to ], yttrium, which is trivalent, is of similar ionic size to ] and its lanthanide neighbors. Due to the relatively gradual decrease in ionic size with increasing atomic number, the rare earth elements have always been difficult to separate. Even with eons of geological time, geochemical separation of the lanthanides has only rarely progressed much farther than a broad separation between light versus heavy lanthanides, otherwise known as the ] and yttrium earths. This geochemical divide is reflected in the first two rare earths that were discovered, ] in 1794 and ] in 1803. As originally found, each comprised the entire mixture of the associated earths. Rare earth minerals, as found, usually are dominated by one group or the other, depending upon which size-range best fits the structural lattice. Thus, among the anhydrous rare earth phosphates, it is the tetragonal mineral ] that incorporates yttrium and the yttrium earths, whereas the monoclinic ] phase incorporates cerium and the cerium earths preferentially. The smaller size of the yttrium group allows it a greater solid solubility in the rock-forming minerals that comprise the Earth's mantle, and thus yttrium and the yttrium earths show less enrichment in the Earth's crust, relative to chondritic abundance, than does cerium and the cerium earths. This has economic consequences: large orebodies of the cerium earths are known around the world, and are being actively exploited. Corresponding orebodies for yttrium tend to be rarer, smaller, and less concentrated. Most of the current supply of yttrium originates in the "ion adsorption clay" ores of Southern China. Some versions of these provide concentrates containing about 65% yttrium oxide, with the heavy lanthanides being present in ratios reflecting the ]: even-numbered heavy lanthanides at abundances of about 5% each, and odd-numbered lanthanides at abundances of about 1% each. Similar compositions are found in xenotime or gadolinite. | Due to ], yttrium, which is trivalent, is of similar ionic size to ] and its lanthanide neighbors. Due to the relatively gradual decrease in ionic size with increasing atomic number, the rare earth elements have always been difficult to separate. Even with eons of geological time, geochemical separation of the lanthanides has only rarely progressed much farther than a broad separation between light versus heavy lanthanides, otherwise known as the ] and yttrium earths. This geochemical divide is reflected in the first two rare earths that were discovered, ] in 1794 and ] in 1803. As originally found, each comprised the entire mixture of the associated earths. Rare earth minerals, as found, usually are dominated by one group or the other, depending upon which size-range best fits the structural lattice. Thus, among the anhydrous rare earth phosphates, it is the tetragonal mineral ] that incorporates yttrium and the yttrium earths, whereas the monoclinic ] phase incorporates cerium and the cerium earths preferentially. The smaller size of the yttrium group allows it a greater solid solubility in the rock-forming minerals that comprise the Earth's mantle, and thus yttrium and the yttrium earths show less enrichment in the Earth's crust, relative to chondritic abundance, than does cerium and the cerium earths. This has economic consequences: large orebodies of the cerium earths are known around the world, and are being actively exploited. Corresponding orebodies for yttrium tend to be rarer, smaller, and less concentrated. Most of the current supply of yttrium originates in the "ion adsorption clay" ores of Southern China. Some versions of these provide concentrates containing about 65% yttrium oxide, with the heavy lanthanides being present in ratios reflecting the ]: even-numbered heavy lanthanides at abundances of about 5% each, and odd-numbered lanthanides at abundances of about 1% each. Similar compositions are found in xenotime or gadolinite. |
Revision as of 11:58, 16 October 2010

As defined by IUPAC, rare earth elements or rare earth metals are a collection of seventeen chemical elements in the periodic table, namely scandium, yttrium, and the fifteen lanthanides. Scandium and yttrium are considered rare earths since they tend to occur in the same ore deposits as the lanthanides and exhibit similar chemical properties.
The term "rare earth" is the English translation of French terre rare (in this case, terre refers to an oxyde). It arises from the rare earth minerals from which they were first isolated, which were uncommon oxide-type minerals (earths) found in Gadolinite extracted from one mine in the village of Ytterby, Sweden. However, with the exception of the highly-unstable promethium, rare earth elements are found in relatively high concentrations in the earth's crust, with cerium being the 25th most abundant element in the Earth's crust at 68 parts per million.
List
A table listing the seventeen rare earth elements, their atomic number and symbol, the etymology of their names, and their main usages is provided here. Some of the rare earths are named for the scientists who discovered or elucidated their elemental properties, and for their geographical discovery.
Z | Symbol | Name | Etymology | Selected Usages |
---|---|---|---|---|
21 | Sc | Scandium | from Latin Scandia (Scandinavia), where the first rare earth ore was discovered. | Aluminium-scandium alloy |
39 | Y | Yttrium | for the village of Ytterby, Sweden, where the first rare earth ore was discovered. | YAG garnet, YBCO high-temperature superconductors |
57 | La | Lanthanum | from the Greek "lanthanon", meaning I am hidden. | High refractive index glass, flint, hydrogen storage, battery-electrodes, camera lenses |
58 | Ce | Cerium | for the dwarf planet Ceres. | Chemical oxidizing agent, polishing powder, yellow colors in glass and ceramics, catalyst for self-cleaning ovens, etc. |
59 | Pr | Praseodymium | from the Greek "praso", meaning leek-green, and "didymos", meaning twin. | Rare-earth magnets, lasers, green colors in glass and ceramics, flint |
60 | Nd | Neodymium | from the Greek "neo", meaning new-one, and "didymos", meaning twin. | Rare-earth magnets, lasers, violet colors in glass and ceramics, ceramic capacitors |
61 | Pm | Promethium | for the Titan Prometheus, who brought fire to mortals. | Nuclear batteries |
62 | Sm | Samarium | for Vasili Samarsky-Bykhovets, who discovered the rare earth ore samarskite. | Rare-earth magnets, lasers, neutron capture, masers |
63 | Eu | Europium | for the continent of Europe. | Red and blue phosphors, lasers, mercury-vapor lamps |
64 | Gd | Gadolinium | for Johan Gadolin (1760–1852), to honor his investigation of rare earths. | Rare-earth magnets, high refractive index glass or garnets, lasers, x-ray tubes, computer memories, neutron capture |
65 | Tb | Terbium | for the village of Ytterby, Sweden. | Green phosphors, lasers, fluorescent lamps |
66 | Dy | Dysprosium | from the Greek "dysprositos", meaning hard to get. | Rare-earth magnets, lasers |
67 | Ho | Holmium | for Stockholm (in Latin, "Holmia"), native city of one of its discoverers. | Laser |
68 | Er | Erbium | for the village of Ytterby, Sweden. | Lasers, vanadium steel |
69 | Tm | Thulium | for the mythological land of Thule. | Portable X-ray machines |
70 | Yb | Ytterbium | for the village of Ytterby, Sweden. | Infrared lasers, chemical reducing agent |
71 | Lu | Lutetium | for Lutetia, the city which later became Paris. |
Abbreviations
The following abbreviations are often used:
- RE = rare earth
- REM = rare earth metals
- REE = rare earth elements
- REO = rare earth oxides
- LREE = light rare earth elements (La-Sm)
- HREE = heavy rare earth elements (Eu-Lu)
Discovery and early history
Rare earth elements became known to the world with the discovery of the black mineral ytterbite (also known as gadolinite) by Lieutenant Carl Axel Arrhenius in the year 1787, in a quarry in the village of Ytterby, Sweden.
The ytterbite, renamed to gadolinite in 1800, of Lt. Arrhenius reached Johann Gadolin, a University of Turku professor, and his analysis yielded an unknown oxide (earth) which he called Ytteria. Anders Gustav Ekeberg isolated beryllium from the gadolinite but failed to recognize other elements which the ore contained. After this discovery in 1794 a mineral from Bastnäs near Riddarhyttan, Sweden, which was believed to be an iron-tungsten mineral, was re-examined by Jöns Jacob Berzelius and Wilhelm Hisinger. In 1803 they obtained a white oxide and called it ceria. Martin Heinrich Klaproth independently discovered the same oxide and called it ochroia.
Thus by 1803 there were two known rare earth elements, yttrium and cerium, although it took another 30 years for researchers to determine that other elements were contained in the two ores ceria and ytteria (the similarity of the rare earth metals' chemical properties made their separation difficult).
In 1839 Carl Gustav Mosander, an assistant of Berzelius, separated ceria by heating the nitrate and dissolving the product in nitric acid. He called the oxide of the soluble salt lanthana. It took him three more years to separate the lanthana further into didymia and pure lanthana. Didymia, although not further separable by Mosander's techniques was a mixture of oxides.
In 1842 Mosander also separated the ytteria into three oxides: pure ytteria, terbia and erbia (all the names are derived from the town name "Ytterby"). The earth giving pink salts he called terbium; the one which yielded yellow peroxide he called erbium.
So in 1842 the number of rare earth elements had reached six: yttrium, cerium, lanthanum, didymium, erbium and terbium.
Nils Johan Berlin and Marc Delafontaine tried also to separate the crude ytteria and found the same substances that Mosander obtained, but Berlin named (1860) the substance giving pink salts erbium and Delafontaine named the substance with the yellow peroxide terbium. This confusion led to several false claims of new elements, such as the mosandrium of J. Lawrence Smith, or the philippium and decipium of Delafontaine.
There were no further discoveries for 30 years, and the element didymium was listed in the periodic table of elements with a molecular mass of 138. In 1879 Delafontaine used the new technique of optical flame spectroscopy and found new spectral lines in didymia, and also in 1879 the new element samarium was isolated by Paul Émile Lecoq de Boisbaudran from the mineral samarskite.
The samaria earth was further separated by Lecoq de Boisbaudran in 1886 and a similar result was obtained by Jean-Charles Galissard de Marignac by direct isolation from samarskithe. They named the element gadolinium after Johan Gadolin, and the oxide was gadolinia.
Further spectroscopic analysis between 1886 and 1901 of samaria, ytteria and samarskite by William Crookes, Lecoq de Boisbaudran and Eugène-Anatole Demarçay yielded new spectroscopic lines indicating an unknown element. Fractional crystallization yielded europium in 1901.
In 1839 the third source for rare earths became available, a mineral similar to gadolinite, uranotantalum (now samarskite). This mineral from Miass in the southern Ural Mountains was described by Gustave Rose. The Russian chemist R. Harmann postulated the new element ilmenium must be present in the mineral, but later Christian Wilhelm Blomstrand, Jean Charles Galissard de Marignac, and Heinrich Rose only found tantalum and niobium.
The exact number of rare earth elements was unclear and a maximum number of 25 was estimated. The use of x-ray spectra (obtained by diffraction in crystals) of Henry Moseley made it possible to determine the atomic numbers. The absolute number of lanthanides had to be 15, with a still missing element 61.
Using this technique Moseley proved that hafnium was not a rare earth element and that the claims of Georges Urbain of having discovered element 72 were false.
In the 1940s Frank Spedding developed an ion exchange procedure for separating and purifying the rare earth elements.
The principal sources of rare earth elements are the minerals bastnäsite, monazite, and loparite and the lateritic ion-adsorption clays. Despite their high relative abundance, rare earth minerals are more difficult to mine and extract than equivalent sources of transition metals (due in part to their similar chemical properties), making the rare earth elements relatively expensive. Their industrial use was very limited until efficient separation techniques were developed, such as ion exchange, fractional crystallization and liquid-liquid extraction during the late 1950s and early 1960s.
Geological distribution
The rare earth elements are often found together. However, Promethium, the only radioactive and hence unstable element, has a very short half life of 20 years and only exists in nature in negligible amounts (approximately 572 g in the earth's crust).
Due to lanthanide contraction, yttrium, which is trivalent, is of similar ionic size to dysprosium and its lanthanide neighbors. Due to the relatively gradual decrease in ionic size with increasing atomic number, the rare earth elements have always been difficult to separate. Even with eons of geological time, geochemical separation of the lanthanides has only rarely progressed much farther than a broad separation between light versus heavy lanthanides, otherwise known as the cerium and yttrium earths. This geochemical divide is reflected in the first two rare earths that were discovered, yttria in 1794 and ceria in 1803. As originally found, each comprised the entire mixture of the associated earths. Rare earth minerals, as found, usually are dominated by one group or the other, depending upon which size-range best fits the structural lattice. Thus, among the anhydrous rare earth phosphates, it is the tetragonal mineral xenotime that incorporates yttrium and the yttrium earths, whereas the monoclinic monazite phase incorporates cerium and the cerium earths preferentially. The smaller size of the yttrium group allows it a greater solid solubility in the rock-forming minerals that comprise the Earth's mantle, and thus yttrium and the yttrium earths show less enrichment in the Earth's crust, relative to chondritic abundance, than does cerium and the cerium earths. This has economic consequences: large orebodies of the cerium earths are known around the world, and are being actively exploited. Corresponding orebodies for yttrium tend to be rarer, smaller, and less concentrated. Most of the current supply of yttrium originates in the "ion adsorption clay" ores of Southern China. Some versions of these provide concentrates containing about 65% yttrium oxide, with the heavy lanthanides being present in ratios reflecting the Oddo-Harkins rule: even-numbered heavy lanthanides at abundances of about 5% each, and odd-numbered lanthanides at abundances of about 1% each. Similar compositions are found in xenotime or gadolinite.
Well-known minerals that contain yttrium include gadolinite, xenotime, samarskite, euxenite, fergusonite, yttrotantalite, yttrotungstite, yttrofluorite (a variety of fluorite), thalenite, yttrialite. Small amounts occur in zircon, which derives its typical yellow fluorescence from some of the accompanying heavy lanthanides. The zirconium mineral eudialyte, such as is found in southern Greenland, also contains small but potentially useful amounts of yttrium. Of the above yttrium minerals, most played a part in providing research quantities of lanthanides during the discovery days. Xenotime is occasionally recovered as a byproduct of heavy sand processing, but has never been nearly as abundant as the similarly recovered monazite (which typically contains a few percent of yttrium). Uranium ores processed in Ontario have occasionally yielded yttrium as a byproduct.
Well-known minerals that contain cerium and the light lanthanides include bastnaesite, monazite, allanite, loparite, ancylite, parisite, lanthanite, chevkinite, cerite, stillwellite, britholite, fluocerite, and cerianite. Over the years, monazite (marine sands from Brazil, India, or Australia; rock from South Africa), bastnaesite (from Mountain Pass California, or several localities in China), and loparite (Kola Peninsula, Russia) have been the principal ores of cerium and the light lanthanides.
Technological applications
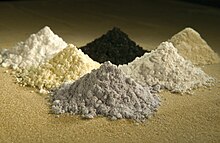
The use of rare earth elements in modern technology has increased dramatically over the past years. Rare earth elements are now incorporated into many technological devices, including superconductors, samarium-cobalt and neodymium-iron-boron high-flux rare-earth magnets, electronic polishers, refining catalysts and hybrid car components (primarily batteries and magnets). Rare earth ions are used as the active ions in luminescent materials used in optoelectronics applications, most notably the Nd:YAG laser. Erbium-doped fiber amplifiers are significant devices in optical-fiber communication systems. Phosphors with rare earth dopants are also widely used in cathode ray tube technology such as television sets. The earliest color television CRTs had a poor-quality red; europium as a phosphor dopant made good red phosphors possible. Yttrium iron garnet (YIG) spheres have been useful as tunable microwave resonators. Rare earth oxides are mixed with tungsten to improve its high temperature properties for welding, replacing thorium, which was mildly hazardous to work with.
Global rare earth production

Until 1948, most of the world's rare earths were sourced from placer sand deposits in India and Brazil. Through the 1950s, South Africa took the status as the world's rare earth source, after large rare earth bearing veins were discovered in Monazite. Through the 1960s until the 1980s, the Mountain Pass rare earth mine in California was the leading producer. Today, the Indian and South African deposits still produce some rare earth concentrates, but they are dwarfed by the scale of Chinese production. China now produces over 97% of the world's rare earth supply, mostly in Inner Mongolia. All of the world's heavy rare earths (such as dysprosium) are come from Chinese rare earth sources such as the polymetallic Bayan Obo deposit.
New demand has recently strained supply, and there is growing concern that the world may soon face a shortage of the rare earths. In several years, worldwide demand for rare earth elements is expected to exceed supply by 40,000 tonnes annually unless major new sources are developed. Additionally, concerns have mounted about the reliability of China as a primary supplier of rare earths. On Sept. 1, 2009, China announced plans to reduce its export quota to 35,000 tons per year in 2010-2015 to conserve scarce resources and protect the environment. Chinese export quotas have also resulted in a dramatic shift in the world's rare earth knowledge base. For example, the division of General Motors which deals with miniaturized magnet research shut down its US office and moved all of its staff to China in 2006. In May 2010, China announced a major, five-month crackdown on illegal mining in order to protect the environment and its resources. This campaign is expected to be concentrated in the South, where most mines are smaller. Illegal rare earth mines are common in rural China and are often known to release toxic wastes into the general water supply. Moreover, in response to the continued detainment of a Chinese fishing boat captain by the Japanese Coast Guard, China instituted an export ban on rare earth shipments to Japan on 22 September 2010, according to the New York Times. Although the Wall Street Journal reports that China has denied the export ban, Japan's former foreign minister Katsuya Okada raised a protest against the effect of China's sudden action on worldwide production.
Spurred by increased demand and concern over China's effective control of the rare earth market, searches for alternative sources in Australia, Brazil, Canada, South Africa and the United States are ongoing. Mines in these countries were closed when China undercut world prices in the 1990s, and it will take a few years to restart production. One example is the Mountain Pass mine in California, which is projected to reopen in 2011. Other significant sites under development outside of China include the Nolans Project in Central Australia, the remote Hoidas Lake project in northern Canada, and the Mount Weld project in Australia. The Hoidas Lake project has the potential to supply about 10% of the $1 billion of REE consumption that occurs in North America every year.
Also under consideration for mining are a sites such at Thor Lake in the Northwest Territories, various locations in Vietnam,, and a site in Southeast Nebraska, where Quantum Rare Earth Development, a Canadian company, is currently conducting test drilling and economic feasibility studies toward opening a niobium mine.
Another recently developed source of rare earths is discarded electronics and other wastes that have significant rare earth components. New advances in recycling technology have made extraction of rare earths from these materials more feasible, and recycling plants are currently operating in Japan, where there is an estimated 300,000 tons of rare earths stored in unused electronics.
See also
References
- Edited by N G Connelly and T Damhus (with R M Hartshorn and A T Hutton), ed. (2005). Nomenclature of Inorganic Chemistry: IUPAC Recommendations 2005 (PDF). Cambridge: RSC Publ. ISBN 0-85404-438-8. Retrieved 2007-12-17.
{{cite book}}
:|editor=
has generic name (help) - Gschneidner KA, Cappellen, ed. (1987). "1787-1987 Two hundred Years of Rare Earths". Rare Earth Information Center, IPRT, North-Holland. IS-RIC 10.
- Spedding F, Daane AH: "The Rare Earths", John Wiley & Sons, Inc., 1961
- P. Belli, R. Bernabei, F. Cappella, R. Cerulli, C.J. Dai, F.A. Danevich, A. d’Angelo, A. Incicchitti, V.V. Kobychev, S.S. Nagorny, S. Nisi, F. Nozzoli, D. Prosperi, V.I. Tretyak, S.S. Yurchenko (2007). "Search for α decay of natural Europium". Nuclear Physics A. 789: 15–29. doi:10.1016/j.nuclphysa.2007.03.001.
{{cite journal}}
: Cite has empty unknown parameter:|month=
(help)CS1 maint: multiple names: authors list (link) - "News and events". US Department of Agriculture. Retrieved 2009-06-06.
- ^ "Haxel G, Hedrick J, Orris J. 2006. Rare earth elements critical resources for high technology. Reston (VA): United States Geological Survey. USGS Fact Sheet: 087‐02" (PDF). Retrieved 2008-04-19.
- ^ ER, Rose. Rare Earths of the Grenville Sub‐Province Ontatio and Quebec. GSC Report Number 59‐10. Ottawa: Geological Survey of Canada Department of Mines and Technical Surveys, 1960.
- ^ China's Rare Earth Dominance, Wikinvest. Retrieved on 11 Aug 2010.
- Chao ECT, Back JM, Minkin J, Tatsumoto M, Junwen W, Conrad JE, McKee EH, Zonglin H, Qingrun M. "Sedimentary carbonate‐hosted giant Bayan Obo REE‐Fe‐Nb ore deposit of Inner Mongolia, China; a cornerstone example for giant polymetallic ore deposits of hydrothermal origin." 1997. United States Geological Survey Publications Warehouse. 29 February 2008 http://pubs.usgs.gov/bul/b2143/
- "Cox C. 2008. Rare earth innovation. Herndon (VA): The Anchor House Inc;". Retrieved 2008-04-19.
- ^ http://www.reuters.com/article/newsOne/idUSTRE57U02B20090831 As hybrid cars gobble rare metals, shortage looms, Aug 31, 2009
- China To Limit Rare Earths Exports, Manufacturing.net, 1 September 2009. Retrieved 2010-08-30.
- C, Cox. "Rare earth innovation: the silent shift to China." 16 November 2006. The Anchor House: Research on Rare Earth Elements. 29 February 2008 http://theanchorhouse.com/2006/11/
- Govt cracks whip on rare earth mining. China Daily, May 21, 2010. Accessed June 3rd, 2010.
- Y, Lee. "South China Villagers Slam Pollution From Rare Earth Mine." 22 February 2008. RFA English Website. 16 March 2008
- BRADSHER, KEITH (2010-09-22). "Amid Tension, China Blocks Vital Exports to Japan". The New York Times Company. Retrieved 22 September 2010.
- JAMES T. AREDDY, DAVID FICKLING And NORIHIKO SHIROUZU (2010-09-23). "China Denies Halting Rare-Earth Exports to Japan". Wall Street Journal. Retrieved 22 September 2010.
- Backlash over China curb on metal exports, Daily Telegraph, London, 29 Aug 2010. Retrieved 2010-08-30.
- "Canadian Firms Step Up Search for Rare-Earth Metals". NYTimes.com. Reuters. 2009-09-09. Retrieved 2009-09-15.
- Leifert, H. Restarting U.S. rare earth production?. Earth magazine. June 2010. Pgs 20-21.
- "Lunn J. 2006. Great western minerals. London: Insigner Beaufort Equity Research" (PDF). Retrieved 2008-04-19.
- "California mine digs in for 'green' gold rush". Reuters. 2009-08-31. Retrieved 2010-03-22.
- Leifert, H. Restarting U.S. rare earth production?. Earth magazine. June 2010. Pgs 20-21.
- "Hoidas Lake Project". Retrieved 2008-09-24.
- "High-tech buried treasure". Retrieved 2010-05-05.
- Tabuchi, Hiroko. "Japan Recycles Minerals From Used Electronics". New York Times. October 5, 2010.
External links
- Tabuchi, Hiroko (5 October 2010). "Japan Recycles Rare Earth Minerals From Used Electronics". The New York Times.
- Kan, Michael (7 October 2010). "Common gadgets may be affected by shortage of rare earths". New Zealand PC World Magazine. Retrieved 6 October 2010.
- Auslin, Michael (13 October 2010). "Japan's Rare-Earth Jolt". Wall Street Journal. Retrieved 13 October 2010.
Periodic table | |||||||||||||||||||
---|---|---|---|---|---|---|---|---|---|---|---|---|---|---|---|---|---|---|---|
Periodic table forms | |||||||||||||||||||
Sets of elements |
| ||||||||||||||||||
Elements | |||||||||||||||||||
History | |||||||||||||||||||
See also | |||||||||||||||||||