Revision as of 04:33, 26 February 2009 editMarek69 (talk | contribs)Autopatrolled, Extended confirmed users, File movers, Pending changes reviewers, Rollbackers195,899 editsm Reverted edits by 69.242.184.230 to last revision by 98.229.90.221 (HG)← Previous edit | Latest revision as of 22:25, 23 December 2024 edit undoNatchouf (talk | contribs)4 edits removed "; in Earth's case, this protects living organisms from harm", as there is no evidence for that. If there is, please provide a reference. | ||
(490 intermediate revisions by more than 100 users not shown) | |||
Line 1: | Line 1: | ||
{{short description|Region around an astronomical object in which its magnetic field affects charged particles}} | |||
{{Mergefrom|Magnetosphere particle motion|date=December 2008}} | |||
] of the magnetosphere of the Earth.]] | |||
'''{{portalpar|Atmospheric sciences}} | |||
In ] and ], a '''magnetosphere''' is a region of space surrounding an ] in which ]s are affected by that object's ].<ref name=NASA>{{cite web | |||
A '''magnetosphere''' is a highly magnetized region around and possessed by an ]. ] is surrounded by a magnetosphere, as are the magnetized planets ], ], ], ], ] and ]. Jupiter's moon ] is magnetized, but too weak to trap solar wind plasma. ] has patchy surface magnetization. The term "magnetosphere" has also been used to describe regions dominated by the magnetic fields of celestial objects, e.g. pulsar magnetospheres. | |||
|title=Magnetospheres | |||
] | |||
|url=https://science.nasa.gov/heliophysics/focus-areas/magnetosphere-ionosphere/ | |||
== History of magnetospheric physics == | |||
|website=NASA Science | |||
{{main|Magnetosphere history}} | |||
|publisher=NASA}}</ref><ref name=Ratcliffe>{{cite book | |||
|last=Ratcliffe|first=John Ashworth| | |||
title=An Introduction to the Ionosphere and Magnetosphere | |||
|date=1972 | |||
|publisher=] | |||
|isbn=9780521083416 | |||
|url=https://archive.org/details/introductiontoio0000ratc|url-access=registration}}</ref> It is created by a ] with an active interior ]. | |||
In the space environment close to a planetary body with a ] such as Earth, the field lines resemble a simple ]. Farther out, ]s can be significantly distorted by the flow of ] ], as emitted from the Sun (i.e., the ]) or a nearby star.<ref name="Britannica">{{cite encyclopedia | |||
The Earth's magnetosphere was discovered in 1958 by ] during the research performed for the ]. Before this, scientists knew that electric currents existed in space, because solar eruptions sometimes led to "magnetic storm" disturbances. No one knew, however, where those currents were and why, or that the ] existed. In August and September of 1958, ] was performed to test a theory about the formation of radiation belts that may have tactical use in war. | |||
|date=2012 | |||
|title=Ionosphere and magnetosphere | |||
|encyclopedia=Encyclopædia Britannica | |||
|publisher=] | |||
|url=https://www.britannica.com/EBchecked/topic/1369043/ionosphere-and-magnetosphere}}</ref><ref name="Van Allen">{{cite book | |||
|last=Van Allen|first=James Alfred| | |||
title=Origins of Magnetospheric Physics | |||
|date=2004 | |||
|publisher=] | |||
|location=Iowa City, Iowa USA|isbn=9780877459217|oclc=646887856}}</ref> Planets having active magnetospheres, like the Earth, are capable of mitigating or blocking the effects of ] or ]. Interactions of particles and atmospheres with magnetospheres are studied under the specialized scientific subjects of ], ], and ]. | |||
==History== | |||
In 1959 ] proposed the name "magnetosphere", when he wrote: | |||
{{main|Magnetosphere chronology}} | |||
Study of Earth's magnetosphere began in 1600, when ] discovered that the magnetic field on the surface of Earth resembled that of a ], a small, magnetized sphere. In the 1940s, ] proposed the model of ], which attributes ] to the motion of Earth's ] ]. Through the use of ]s, scientists were able to study the variations in Earth's magnetic field as functions of both time and latitude and longitude. | |||
Beginning in the late 1940s, rockets were used to study ]. In 1958, ], the first of the Explorer series of space missions, was launched to study the intensity of cosmic rays above the atmosphere and measure the fluctuations in this activity. This mission observed the existence of the ] (located in the inner region of Earth's magnetosphere), with the follow-up ] later that year definitively proving its existence. Also during 1958, ] proposed the idea of the ], with the term 'magnetosphere' being proposed by ] in 1959 to explain how the solar wind interacted with the Earth's magnetic field. The later mission of Explorer 12 in 1961 led by the Cahill and Amazeen observation in 1963 of a sudden decrease in magnetic field strength near the noon-time meridian, later was named the ]. By 1983, the ] observed the magnetotail, or the distant magnetic field.<ref name="Van Allen"/> | |||
:"The region above the ionosphere in which the magnetic field of the earth has a dominant control over the motions of gas and fast charged particles is known to extend out to a distance of the order of 10 earth radii; it may appropriately be called the magnetosphere . | |||
==Structure and behavior== | |||
== Earth's magnetosphere == | |||
Magnetospheres are dependent on several variables: the type of astronomical object, the nature of sources of plasma and momentum, the ] of the object's spin, the nature of the axis about which the object spins, the axis of the magnetic dipole, and the magnitude and direction of the flow of ]. | |||
] flows from left to right.]] | |||
{{main|Earth's magnetic field}} | |||
The planetary distance where the magnetosphere can withstand the solar wind pressure is called the Chapman–Ferraro distance. This is usefully modeled by the formula wherein <math>R_{\rm P}</math> represents the radius of the planet, <math>B_{\rm surf}</math> represents the magnetic field on the surface of the planet at the equator, and <math>V_{\rm SW}</math> represents the velocity of the solar wind: | |||
The magnetosphere of Earth is a region in space whose shape is determined by the extent of Earth's internal magnetic field, the ] plasma, and the ] (IMF). In the magnetosphere, a mix of free ] and ] from both the solar wind and the Earth's ionosphere is confined by magnetic and electric forces that are much stronger than gravity and collisions. In spite of its name, the magnetosphere is distinctly non-spherical. On the side facing the Sun, the distance to its boundary (which varies with solar wind intensity) is about 70,000 km (10-12 Earth radii or R<sub>E</sub>, where 1 R<sub>E</sub>=6371 km; unless otherwise noted, all distances here are from the Earth's center). The boundary of the magnetosphere ("]") is roughly bullet shaped, about 15 R<sub>E</sub> abreast of Earth and on the night side (in the "magnetotail" or "geotail") approaching a cylinder with a radius 20-25 R<sub>E</sub>. The tail region stretches well past 200 R<sub>E</sub>, and the way it ends is not well-known. | |||
:<math>R_{\rm CF}=R_{\rm P} \left( \frac{B_{\rm surf}^2}{\mu_{0} \rho V_{\rm SW}^2} \right) ^{\frac{1}{6}}</math> | |||
The outer neutral gas envelope of Earth, or ], consists mostly of the lightest atoms, hydrogen and helium, and continues beyond 4-5 R<sub>E</sub>, with diminishing density. The hot plasma ions of the magnetosphere acquire electrons during collisions with these atoms and create an escaping "glow" of fast atoms that have been used to image the hot plasma clouds by the ] mission. The upward extension of the ionosphere, known as the ], also extends beyond 4-5 R<sub>E</sub> with diminishing density, beyond which it becomes a flow of light ions called the ] that escapes out of the magnetosphere into the solar wind. Energy deposited in the ionosphere by ] strongly heats the heavier atmospheric components such as oxygen and ]s of oxygen and nitrogen, which would not otherwise escape from Earth's gravity. Owing to this highly variable heating, however, a heavy atmospheric or ionospheric outflow of plasma flows during disturbed periods from the auroral zones into the magnetosphere, extending the region dominated by terrestrial material, known as the fourth or plasma ], at times out to the ]. | |||
A magnetosphere is classified as "intrinsic" when <math>R_{\rm CF} \gg R_{\rm P}</math>, or when the primary opposition to the flow of solar wind is the magnetic field of the object. ], Earth, ], ], ], ], and ], for example, exhibit intrinsic magnetospheres. A magnetosphere is classified as "induced" when <math>R_{\rm CF} \ll R_{\rm P}</math>, or when the solar wind is not opposed by the object's magnetic field. In this case, the solar wind interacts with the atmosphere or ionosphere of the planet (or surface of the planet, if the planet has no atmosphere). ] has an induced magnetic field, which means that because Venus appears to have no ], the only magnetic field present is that formed by the solar wind's wrapping around the physical obstacle of Venus (see also ]). When <math>R_{\rm CF} \approx R_{\rm P}</math>, the planet itself and its magnetic field both contribute. It is possible that ] is of this type.<ref>{{cite journal|last1=Blanc|first1=M.|last2=Kallenbach|first2=R.|last3=Erkaev|first3=N.V.|title=Solar System Magnetospheres|journal=Space Science Reviews|volume=116|date=2005|issue=1–2|pages=227–298|doi=10.1007/s11214-005-1958-y|bibcode=2005SSRv..116..227B |s2cid=122318569}}</ref> | |||
== General properties == | |||
" (1966) ''Astrophysics and Space Science'', v. 242, Issue 1/2, p. 93-163.</ref>]] | |||
Two factors determine the structure and behavior of the magnetosphere: (1) The internal field of the Earth, and (2) The solar wind. | |||
# The ] of the Earth (its "main field") appears to be generated in the ] by a ], associated with the circulation of liquid metal in the core, driven by internal heat sources. Its major part resembles the field of a bar magnet ("dipole field") inclined by about 10° to the rotation axis of Earth, but more complex parts ("higher harmonics") also exist, as first shown by ]. The dipole field has an intensity of about 30,000-60,000 ] (nT) at the Earth's surface, and its intensity diminishes like the inverse of the cube of the distance, i.e. at a distance of ''R'' Earth radii it only amounts to 1/''R''³ of the surface field in the same direction. Higher harmonics diminish faster, like higher powers of 1/''R'', making the dipole field the only important internal source in most of the magnetosphere. | |||
# ] is a fast outflow of hot plasma from the sun in all directions. Above the sun's equator it typically attains 400 km/s; above the sun's poles, up to twice as much. The flow is powered by the million-degree temperature of the sun's ], for which no generally accepted explanation exists as yet. Its composition resembles that of the Sun—about 95% of the ions are protons, about 4% helium nuclei, with 1% of heavier matter (], ], ], ], ], ]... up to ]) and enough electrons to keep charge neutrality. At Earth's orbit its typical density is 6 ions/cm<SUP>3</SUP> (variable, as is the velocity), and it contains a variable ] (IMF) of (typically) 2–5 nT. The IMF is produced by stretched-out magnetic field lines originating on the Sun, a process described in the article ]. | |||
==Structure== | |||
Physical reasons make it difficult for solar wind plasma with its embedded IMF to mix with terrestrial plasma whose magnetic field has a different source. The two plasmas end up separated by a boundary, the magnetopause, and the Earth's plasma is confined to a cavity inside the flowing solar wind, the magnetosphere. The isolation is not complete, thanks to secondary processes such as ] —otherwise it would be hard for the solar wind to transmit much energy to the magnetosphere—but it still determines the overall configuration. | |||
] | |||
===Bow shock=== | |||
An additional feature is a collision-free ] which forms in the solar wind ahead of Earth, typically at 13.5 ''R''<SUB>E</SUB> on the sunward side. It forms because the solar velocity of the wind exceeds (typically 2–3 times) that of ], a family of characteristic waves with which disturbances propagate in a magnetized fluid. In the region behind the shock ("magnetosheath") the velocity drops briefly to the Alfvén velocity (and the temperature rises, absorbing lost kinetic energy), but the velocity soon rises back as plasma is dragged forward by the surrounding solar wind flow. | |||
] and artist's concept of the bow shock around ]]] | |||
{{main|Bow shock}} | |||
The bow shock forms the outermost layer of the magnetosphere; the boundary between the magnetosphere and the ambient medium. For stars, this is usually the boundary between the ] and ]; for planets, the speed of the solar wind there decreases as it approaches the magnetopause.<ref>{{cite arXiv|last1=Sparavigna|first1=A.C.|last2=Marazzato|first2=R.|title=Observing stellar bow shocks|date=10 May 2010|class=physics.space-ph |eprint=1005.1527}}</ref> Due to interactions with the bow shock, the ] ] gains a substantial ], leading to various ] upstream and downstream of the bow shock. <ref>{{cite journal|last1=Pokhotelov|first1=D.|last2=von Alfthan|first2=S.|last3=Kempf|first3=Y.|last4=Vainio|first4=R.|display-authors=et al. |title= Ion distributions upstream and downstream of the Earth's bow shock: first results from Vlasiator| journal=Annales Geophysicae|date=2013-12-17|volume=31|issue=12 |pages=2207–2212|doi=10.5194/angeo-31-2207-2013|doi-access=free |bibcode=2013AnGeo..31.2207P }}</ref> | |||
===Magnetosheath=== | |||
To understand the magnetosphere, one needs to visualize its ], that everywhere point in the direction of the magnetic field—e.g., diverging out near the magnetic north pole (or geographic southpole), and converging again around the magnetic south pole (or the geographic northpole), where they enter the Earth. They can be visualized like wires which tie the magnetosphere together—wires that also ] of trapped particles, which slide along them like beads (though other motions may also occur). | |||
{{main|Magnetosheath}} | |||
The magnetosheath is the region of the magnetosphere between the bow shock and the magnetopause. It is formed mainly from shocked solar wind, though it contains a small amount of plasma from the magnetosphere.<ref name=cluster>{{Cite book|editor1-last=Paschmann|editor1-first=G.|editor2-last=Schwartz|editor2-first=S.J.|editor3-last=Escoubet|editor3-first=C.P.|editor4-last=Haaland|editor4-first=S.|title=Outer Magnetospheric Boundaries: Cluster Results|journal=Space Science Reviews|date=2005|volume=118|issue=1–4|isbn=978-1-4020-3488-6|doi=10.1007/1-4020-4582-4 |url=https://cds.cern.ch/record/1250411/files/978-1-4020-4582-0_BookTOC.pdf|series=Space Sciences Series of ISSI|bibcode=2005ombc.book.....P }}</ref> It is an area exhibiting high particle ], where the direction and magnitude of the magnetic field varies erratically. This is caused by the collection of solar wind gas that has effectively undergone ]. It acts as a cushion that transmits the pressure from the flow of the solar wind and the barrier of the magnetic field from the object.<ref name="Van Allen"/> | |||
===Magnetopause=== | |||
== Radiation belts == | |||
{{main|Magnetopause}} | |||
When the first scientific satellites were launched in the first half of 1958--Explorers 1 and ] by the US, ] by the Soviet Union--they observed an intense (and unexpected) ] around Earth, held by its magnetic field. "My God, Space is Radioactive!" exclaimed one of ]'s colleagues, when the meaning of those observations was realized. That was the "inner radiation belt" of protons with energies in the range 10-100 MeV (]s), attributed later to "albedo neutron decay," a secondary effect of the interaction of ] with the upper atmosphere. It is centered on field lines crossing the equator about 1.5 ''R''<SUB>E</SUB> from the Earth's center. | |||
The magnetopause is the area of the magnetosphere wherein the pressure from the planetary magnetic field is balanced with the pressure from the solar wind.<ref name=Britannica/> It is the convergence of the shocked solar wind from the magnetosheath with the magnetic field of the object and plasma from the magnetosphere. Because both sides of this convergence contain magnetized plasma, the interactions between them are complex. The structure of the magnetopause depends upon the ] and ] of the plasma, as well as the magnetic field.<ref>{{cite book |chapter=The Magnetopause |last1=Russell |first1=C.T. |editor-last1=Russell |editor-first1=C.T. |editor-last2=Priest |editor-first2=E.R. |editor-last3=Lee |editor-first3=L.C. |title=Physics of magnetic flux ropes |date=1990 |publisher=American Geophysical Union |isbn=9780875900261 |pages=439–453 |url=http://www-ssc.igpp.ucla.edu/ssc/tutorial/magnetopause.html |archive-url=https://web.archive.org/web/19990202125049/http://www-ssc.igpp.ucla.edu/ssc/tutorial/magnetopause.html |archive-date=2 February 1999}}</ref> The magnetopause changes size and shape as the pressure from the solar wind fluctuates.<ref>{{cite web |first1=David P. |last1=Stern |first2=Mauricio |last2=Peredo |title=The Magnetopause |url=https://www-spof.gsfc.nasa.gov/Education/wmpause.html |website=The Exploration of the Earth's Magnetosphere |publisher=NASA |date=20 November 2003 |access-date=19 August 2019 |archive-date=19 August 2019 |archive-url=https://web.archive.org/web/20190819221711/https://www-spof.gsfc.nasa.gov/Education/wmpause.html |url-status=dead }}</ref> | |||
===Magnetotail=== | |||
Later a population of trapped ions and electrons was observed on field lines crossing the equator at 2.5–8 ''R''<SUB>E</SUB>. The high-energy part of that population (about 1 MeV) became known as the "outer radiation belt", but its bulk is at lower energies (peak about 65 keV) and is identified as the ] plasma. | |||
Opposite the compressed magnetic field is the magnetotail, where the magnetosphere extends far beyond the astronomical object. It contains two lobes, referred to as the northern and southern tail lobes. Magnetic field lines in the northern tail lobe point towards the object while those in the southern tail lobe point away. The tail lobes are almost empty, with few charged particles opposing the flow of the solar wind. The two lobes are separated by a plasma sheet, an area where the magnetic field is weaker, and the density of charged particles is higher.<ref name="tail">{{cite web|title=The Tail of the Magnetosphere|url=http://www-spof.gsfc.nasa.gov/Education/wtail.html|publisher=NASA|access-date=22 December 2012|archive-date=7 February 2018|archive-url=https://web.archive.org/web/20180207114437/https://www-spof.gsfc.nasa.gov/Education/wtail.html|url-status=dead}}</ref> | |||
===Earth's magnetosphere{{anchor|Earth}}=== | |||
The trapping of charged particles in a magnetic field can be quite stable. This is particularly true in the inner belt, because the build-up of trapped protons from albedo neutrons is quite slow, requiring years to reach observed intensities. In July 1962, the United States ] high over the South Pacific at around 400 km in the upper atmosphere, in this region, creating an artificial belt of high-energy electrons, and some of them were still around 4–5 years later (such tests are now banned by treaty). | |||
<!-- ] redirects to this section. If this section's name is changed, please update that incoming redirect. Thanks! --> | |||
{{See also|Earth's magnetic field#Magnetosphere|Van Allen radiation belt}} | |||
The outer belt and ring current are less persistent, because charge-exchange collisions with atoms of the geocorona (see above) tends to remove their particles. That suggests the existence of an effective source mechanism, continually supplying this region with fresh plasma. It turns out that the magnetic barrier can be broken down by electric forces, as discussed in MSPF. If plasma is pushed hard enough, it generates ]s which allow it to move in response to the push, often (not always) deforming the magnetic field in the process. | |||
{{further|Plasmasphere}} | |||
] | |||
] | |||
Over Earth's ], the magnetic field lines become almost horizontal, then return to reconnect at high latitudes. However, at high altitudes, the magnetic field is significantly distorted by the solar wind and its solar magnetic field. On the dayside of Earth, the magnetic field is significantly compressed by the solar wind to a distance of approximately {{convert|65000|km|sp=us}}. Earth's bow shock is about {{convert|17|km|sp=us}} thick<ref>{{cite news|title=Cluster reveals Earth's bow shock is remarkably thin|url=http://sci.esa.int/science-e/www/object/index.cfm?fobjectid=49637|newspaper=]|date=16 November 2011}}</ref> and located about {{convert|90000|km|sp=us}} from Earth.<ref>{{cite news|title=Cluster reveals the reformation of Earth's bow shock|url=http://sci.esa.int/science-e/www/object/index.cfm?fobjectid=40994|newspaper=European Space Agency|date=11 May 2011}}</ref> The magnetopause exists at a distance of several hundred kilometers above Earth's surface. Earth's magnetopause has been compared to a ] because it allows solar wind particles to enter. ] occur when large swirls of plasma travel along the edge of the magnetosphere at a different velocity from the magnetosphere, causing the plasma to slip past. This results in ], and as the magnetic field lines break and reconnect, solar wind particles are able to enter the magnetosphere.<ref>{{cite news|title=Cluster observes a 'porous' magnetopause|url=http://sci.esa.int/science-e/www/object/index.cfm?fobjectid=50977|newspaper=European Space Agency|date=24 October 2012}}</ref> On Earth's nightside, the magnetic field extends in the magnetotail, which lengthwise exceeds {{convert|6300000|km|sp=us}}.<ref name=Britannica/> Earth's magnetotail is the primary source of the ].<ref name=tail/> Also, NASA scientists have suggested that Earth's magnetotail might cause "dust storms" on the Moon by creating a potential difference between the day side and the night side.<ref>http://www.nasa.gov/topics/moonmars/features/magnetotail_080416.html {{Webarchive|url=https://web.archive.org/web/20211114122639/https://www.nasa.gov/topics/moonmars/features/magnetotail_080416.html |date=14 November 2021 }} NASA, ''The Moon and the Magnetotail''</ref> | |||
===Other objects=== | |||
== Magnetic tails == | |||
Many astronomical objects generate and maintain magnetospheres. In the Solar System this includes the Sun, ], ], ], ], ], ],<ref name="Planetary Shields: Magnetospheres">{{cite web |title=Planetary Shields: Magnetospheres |url=https://mobile.arc.nasa.gov/public/iexplore/missions/pages/yss/november2011.html |publisher=NASA |access-date=5 January 2020}}</ref> and ]. The ] is the largest planetary magnetosphere in the Solar System, extending up to {{convert|7000000|km|sp=us}} on the dayside and almost to the orbit of ] on the nightside.<ref>{{cite encyclopedia |url=http://www.igpp.ucla.edu/people/mkivelson/Publications/279-Ch24.pdf |title=The configuration of Jupiter's magnetosphere |first=K. K. |last=Khurana |author2=Kivelson, M. G. |display-authors=etal |isbn=978-0-521-81808-7 |encyclopedia=Jupiter: The Planet, Satellites and Magnetosphere |publisher=] |editor=Bagenal, Fran |editor2=Dowling, Timothy E. |editor3=McKinnon, William B. |date=2004 }}</ref> Jupiter's magnetosphere is stronger than Earth's by an ], and its ] is approximately 18,000 times larger.<ref>{{cite journal|last=Russell|first=C.T.|title=Planetary Magnetospheres|journal=Reports on Progress in Physics|volume=56|issue=6|pages=687–732|date=1993|doi=10.1088/0034-4885/56/6/001|bibcode=1993RPPh...56..687R|s2cid=250897924 }}</ref> ], ], and ], on the other hand, have no magnetic field. This may have had significant effects on their geological history. It is theorized that Venus and Mars may have lost their primordial water to ] and the solar wind. A strong magnetosphere greatly slows this process.<ref name="Planetary Shields: Magnetospheres"/><ref>{{cite web |title=X-ray Detection Sheds New Light on Pluto |url=https://www.nasa.gov/mission_pages/chandra/x-ray-detection-sheds-new-light-on-pluto.html |access-date=3 December 2016 |date=14 September 2016 |author=NASA |website=nasa.gov}}</ref> | |||
] | |||
A magnetic tail or magnetotail is formed by pressure from the solar wind on a planet's magnetosphere. The magnetotail can extend great distances away from its originating planet. Earth's magnetic tail extends at least 200 Earth radii in the anti-sunward direction well beyond the orbit of the Moon at about 60 Earth radii, while Jupiter's magnetic tail extends beyond the orbit of Saturn. On occasion Saturn is immersed inside the Jovian magnetosphere. | |||
] | |||
The extended magnetotail results from energy stored in the planet's magnetic field. At times this energy is released and the magnetic field becomes temporarily more ]-like. As it does so that stored energy goes to energize plasma trapped on the involved magnetic field lines. Some of that plasma is driven tailward and into the distant solar wind. The rest is injected into the inner magnetosphere where it results in the aurora and the ring current plasma population. The resulting energetic plasma and electric currents can disrupt spacecraft operations, communication and navigation. | |||
Magnetospheres generated by ]s are thought to be common, though the first discoveries did not come until the 2010s. In 2014, a magnetic field around ] was inferred from the way hydrogen was evaporating from the planet.<ref>{{Cite web|author1=Charles Q. Choi|date=2014-11-20|title=Unlocking the Secrets of an Alien World's Magnetic Field|url=https://www.space.com/27828-alien-planet-magnetic-field-strength.html|access-date=2022-01-17|website=Space.com|language=en}}</ref><ref>{{Cite journal|doi=10.1126/science.1257829|pmid=25414310 |title=Magnetic moment and plasma environment of HD 209458b as determined from Ly observations |journal=Science |volume=346 |issue=6212 |pages=981–984 |year=2014 |last1=Kislyakova |first1=K. G.|last2=Holmstrom |first2=M. |last3=Lammer |first3=H. |last4=Odert |first4=P. |last5=Khodachenko |first5=M. L. |bibcode=2014Sci...346..981K |arxiv = 1411.6875 |s2cid=206560188}}</ref> In 2019, the strength of the surface magnetic fields of 4 ]s were estimated and ranged between 20 and 120 ] compared to Jupiter's surface magnetic field of 4.3 gauss.<ref>{{Cite web|author1=Passant Rabie|date=2019-07-29|title=Magnetic Fields of 'Hot Jupiter' Exoplanets Are Much Stronger Than We Thought|url=https://www.space.com/hot-jupiter-magnetic-fields-measured-for-first-time.html|access-date=2022-01-17|website=Space.com|language=en}}</ref><ref>{{Cite journal|last1=Cauley|first1=P. Wilson|last2=Shkolnik|first2=Evgenya L.|last3=Llama|first3=Joe|last4=Lanza|first4=Antonino F.|date=Dec 2019|title=Magnetic field strengths of hot Jupiters from signals of star-planet interactions|journal=Nature Astronomy|volume=3|issue=12|pages=1128–1134|doi=10.1038/s41550-019-0840-x|arxiv=1907.09068|bibcode=2019NatAs...3.1128C|s2cid=198147426|issn=2397-3366}}</ref> In 2020, a radio emission in the 14-30 MHz band was detected from the ] system, likely associated with ] from the poles of ] a signature of a planetary magnetic field.<ref>{{citation |last1=Turner |first1=Jake D. |title=The search for radio emission from the exoplanetary systems 55 Cancri, υ Andromedae, and τ Boötis using LOFAR beam-formed observations |journal=Astronomy & Astrophysics |volume=645 |pages=A59 |year=2021 |arxiv=2012.07926 |bibcode=2021A&A...645A..59T |doi=10.1051/0004-6361/201937201 |s2cid=212883637 |last2=Zarka |first2=Philippe |last3=Grießmeier |first3=Jean-Mathias |last4=Lazio |first4=Joseph |last5=Cecconi |first5=Baptiste |last6=Emilio Enriquez |first6=J. |last7=Girard |first7=Julien N. |last8=Jayawardhana |first8=Ray |last9=Lamy |first9=Laurent |last10=Nichols |first10=Jonathan D. |last11=De Pater |first11=Imke}}</ref><ref>{{Cite web |last=O'Callaghan |first=Jonathan |date=2023-08-07 |title=Exoplanets Could Help Us Learn How Planets Make Magnetism |url=https://www.quantamagazine.org/exoplanets-could-help-us-learn-how-planets-make-magnetism-20230807/ |access-date=2023-08-07 |website=Quanta Magazine |language=en}}</ref> In 2021 a magnetic field generated by ] became the first to be confirmed.<ref name= sedHatp11b> Signatures of Strong Magnetization and Metal-poor Atmosphere for a Neptune-Size Exoplanet, Ben-Jaffel et al. 2021</ref> The first unconfirmed detection of a magnetic field generated by a terrestrial exoplanet was found in 2023 on ].<ref name="Pineda2023">{{cite journal |last1=Pineda |first1=J. Sebastian |last2=Villadsen |first2=Jackie |date=April 2023 |title=Coherent radio bursts from known M-dwarf planet host YZ Ceti |journal=] |volume=7 |issue= 5|pages=569–578 |doi=10.1038/s41550-023-01914-0 |arxiv=2304.00031 |bibcode=2023NatAs...7..569P}}</ref><ref name="Trigilio2023">{{cite arXiv |last1=Trigilio |first1=Corrado |last2=Biswas |first2=Ayan |display-authors=etal |date=May 2023 |title=Star-Planet Interaction at radio wavelengths in YZ Ceti: Inferring planetary magnetic field |eprint=2305.00809 |class=astro-ph.EP}}</ref><ref>{{Cite web |date=2023-04-10 |title=A magnetic field on a nearby Earth-sized exoplanet? |url=https://earthsky.org/space/magnetic-field-exoplanets-yz-ceti-b/ |access-date=2023-08-07 |website=earthsky.org |language=en-US}}</ref><ref>{{Cite web |last=O'Callaghan |first=Jonathan |date=7 August 2023 |title=Exoplanets Could Help Us Learn How Planets Make Magnetism |url=https://www.quantamagazine.org/exoplanets-could-help-us-learn-how-planets-make-magnetism-20230807/ |website=]}}</ref> | |||
==See also== | |||
== Electric currents in space == | |||
*] | |||
*] | |||
==References== | |||
Magnetic fields in the magnetosphere arise from the ]'s internal magnetic field as well as from electric currents that flow in the magnetospheric plasma: the plasma acts as a kind of ]. Magnetic fields from currents that circulate in the magnetospheric plasma extend the Earth's magnetism much further in space than would be predicted from the Earth's internal field alone. Such currents also determine the field's structure far from Earth, creating the regions described in the introduction above. | |||
{{Reflist}} | |||
Unlike in a conventional resistive electric circuit, where currents are best thought of as arising as a response to an applied voltage, currents in the magnetosphere are better seen as being caused by the structure and motion of the plasma in its associated magnetic field. For instance, electrons and positive ions trapped in the dipole-like field near the Earth tend to circulate around the magnetic axis of the dipole (the line connecting the magnetic poles) in a ring around the Earth, without gaining or losing energy (this is known as ] motion). Viewed from above the magnetic north pole (geographic south), ions circulate clockwise, electrons counterclockwise, producing a net circulating clockwise current, known (from its shape) as the ]. No voltage is needed--the current arises naturally from the motion of the ions and electrons in the magnetic field. | |||
Any such current will modify the magnetic field. The ring current, for instance, strengthens the field on its outside, helping expand the size of the magnetosphere. At the same time, it weakens the magnetic field in its interior. In a magnetic storm, plasma is added to the ring current, making it temporarily stronger, and the field at Earth is observed to weaken by up to 1-2%. | |||
The deformation of the magnetic field, and the flow of electric currents in it, are intimately linked, making it often hard to label one as cause and the other as effect. Frequently (as in the magnetopause and the magnetotail) it is intuitively more useful to regard the distribution and flow of plasma as the primary effect, producing the observed magnetic structure, with the associated electric currents just one feature of those structures, more of a consistency requirement of the magnetic structure. | |||
As noted, one exception (at least) exists, a case where voltages '''do''' drive currents. That happens with ]s, which flow from distant space into the near-polar ionosphere, continue at least some distance in the ionosphere, and then return to space. (Part of the current then detours and leaves Earth again along field lines on the morning side, flows across midnight as part of the ring current, then comes back to the ionosphere along field lines on the evening side and rejoins the pattern.) The full circuit of those currents, under various conditions, is still under debate. | |||
Because the ionosphere is an ohmic conductor of sorts, such flow will heat it up. It will also give rise to secondary Hall currents, and accelerate magnetospheric particles--electrons in the arcs of the ], and singly-ionized oxygen ions (O+) which contribute to the ring current. | |||
== Classification of magnetic fields == | |||
] Regardless of whether they are viewed as sources or consequences of the magnetospheric field structure, electric currents flow in closed circuits. That makes them useful for classifying different parts of the magnetic field of the magnetosphere, each associated with a distinct type of circuit. In this way the field of the magnetosphere is often resolved into 5 distinct parts, as follows. | |||
# The internal field of the Earth ("main field") arising from electric currents in the core. It is dipole-like, modified by higher harmonic contributions. | |||
# The ] field, carried by plasma trapped in the dipole-like field around Earth, typically at distances 3–8 ''R''<SUB>E</SUB> (less during large storms). Its current flows (approximately) around the magnetic equator, mainly clockwise when viewed from north. (A small counterclockwise ring current flows at the inner edge of the ring, caused by the fall-off in plasma density as Earth is approached). | |||
# The field confining the Earth's plasma and magnetic field inside the magnetospheric cavity. The currents responsible for it flow on the magnetopause, the interface between the magnetosphere and the solar wind, described in the introduction. Their flow, again, may be viewed as arising from the geometry of the magnetic field (rather than from any driving voltage), a consequence of "Ampére's law" (embodied in Maxwell's equations) which in this case requires an electric current to flow along any interface between magnetic fields of different directions and/or intensities.] | |||
# The system of tail currents. The magnetotail consists of twin bundles of oppositely directed magnetic field (the "tail lobes"), directed earthwards in the northern half of the tail and away from Earth in the southern half. In between the two exists a layer ("plasma sheet") of denser plasma (0.3-0.5 ions/cm<SUP>3</sup> vs. 0.01-0.02 in the lobes), and because of the difference between the adjoining magnetic fields, by Ampére's law an electric current flows there too, directed from dawn to dusk. The flow closes (as it must) by following the tail magnetopause--part over the northern lobe, part over the southern one. | |||
# The Birkeland current field (and its branches in the ionosphere and ring current), a circuit is associated with the polar aurora. Unlike the 3 preceding current systems, it does require a constant input of energy, to provide the heating of its ionospheric path and the acceleration of auroral electrons and of positive ions. The energy probably comes from a dynamo process, meaning that part of the circuit threads a plasma moving relative to Earth, either in the solar wind and in "boundary layer" flows which it drives just inside the magnetopause, or by plasma moving earthward in the magnetotail, as observed during substorms (below). | |||
== Magnetic substorms and storms == | |||
Earlier it was stated that "if plasma is pushed hard enough, it generates ]s which allow it to move in response to the push, often (not always) deforming the magnetic field in the process." Two examples of such "pushing" are particularly important in the magnetosphere. The ] mission is a ] program to study in detail the physical processes involved in substorms. | |||
The more common one occurs when the north-south component ''B''<SUB>z</SUB> of the interplanetary magnetic field (IMF) is appreciable and points southward. In this state field lines of the magnetosphere are relatively strongly linked to the IMF, allowing energy and plasma to enter it at relatively high rates. This swells up the magnetotail and makes it unstable. Ultimately the tail's structure changes abruptly and violently, a process known as a magnetic substorm. | |||
]"]] One possible scenario (the subject is still being debated) is as follows. As the magnetotail swells, it creates a wider obstacle to the solar wind flow, causing its widening portion to be squeezed more by the solar wind. In the end, this squeezing breaks apart field lines in the plasma sheet ("]"), and the distant part of the sheet, no longer attached to the Earth, is swept away as an independent magnetic structure ("]"). The near-Earth part snaps back earthwards, energizing its particles and producing Birkeland currents and bright auroras. As observed in the 1970s by the ATS satellites at 6.6 ''R''<SUB>E</SUB>, when conditions are favorable that can happen up to several times a day. | |||
Substorms generally do not substantially add to the ring current. That happens in magnetic storms, when following an eruption on the sun (a "coronal mass ejection" or a "solar flare"—details are still being debated, see MSPF) a fast-moving plasma cloud hits the Earth. If the IMF has a southward component, this not only pushes the magnetopause boundary closer to Earth (at times to about half its usual distance), but it also produces an injection of plasma from the tail, much more vigorous than the one associated with substorms. | |||
The plasma population of the ring current may now grow substantially, and a notable part of the addition consists of O+ oxygen ions extracted from the ionosphere as a by-product of the ]. In addition, the ring current is driven earthward (which energizes its particles further), temporarily modifying the field around the Earth and thus shifting the aurora (and its current system) closer to the equator. The magnetic disturbance may decay within 1–3 days as many ions are removed by charge exchange, but the higher energies of the ring current can persist much longer. | |||
== See also == | |||
* ] for applications in ] | |||
* ] | |||
{{Magnetospherics}} | {{Magnetospherics}} | ||
{{In space}} | |||
{{Portal bar|Physics|Astronomy|Stars|Spaceflight|Solar System}} | |||
{{Authority control}} | |||
{{Use dmy dates|date=September 2019}} | |||
] | |||
== References == | |||
] | |||
<references/> | |||
* '']'' by ] (1994) | |||
* ] by ]"(2002)" | |||
* {{cite web | author = D. P. Stern, M. Peredo | date = 2004-09-28 | url = http://www-istp.gsfc.nasa.gov/Education/Intro.html | title = The Exploration of the Earth's Magnetosphere | publisher = NASA | accessdate = 2006-08-22 }} | |||
== External links == | |||
* | |||
* | |||
* | |||
* | |||
* | |||
*"''''" Tool dedicated to the 3d simulation of charged particles in the magnetosphere.. | |||
] | |||
] | ] | ||
] | |||
] | ] | ||
] | ] | ||
] | |||
] | |||
] | |||
] | |||
] | |||
] | |||
] | |||
] | |||
] | |||
] | |||
] | |||
] | |||
] | |||
] | |||
] | |||
] | |||
] | |||
] | |||
] | |||
] | |||
] | |||
] | |||
] | |||
] | |||
] | |||
] |
Latest revision as of 22:25, 23 December 2024
Region around an astronomical object in which its magnetic field affects charged particlesIn astronomy and planetary science, a magnetosphere is a region of space surrounding an astronomical object in which charged particles are affected by that object's magnetic field. It is created by a celestial body with an active interior dynamo.
In the space environment close to a planetary body with a dipole magnetic field such as Earth, the field lines resemble a simple magnetic dipole. Farther out, field lines can be significantly distorted by the flow of electrically conducting plasma, as emitted from the Sun (i.e., the solar wind) or a nearby star. Planets having active magnetospheres, like the Earth, are capable of mitigating or blocking the effects of solar radiation or cosmic radiation. Interactions of particles and atmospheres with magnetospheres are studied under the specialized scientific subjects of plasma physics, space physics, and aeronomy.
History
Main article: Magnetosphere chronologyStudy of Earth's magnetosphere began in 1600, when William Gilbert discovered that the magnetic field on the surface of Earth resembled that of a terrella, a small, magnetized sphere. In the 1940s, Walter M. Elsasser proposed the model of dynamo theory, which attributes Earth's magnetic field to the motion of Earth's iron outer core. Through the use of magnetometers, scientists were able to study the variations in Earth's magnetic field as functions of both time and latitude and longitude.
Beginning in the late 1940s, rockets were used to study cosmic rays. In 1958, Explorer 1, the first of the Explorer series of space missions, was launched to study the intensity of cosmic rays above the atmosphere and measure the fluctuations in this activity. This mission observed the existence of the Van Allen radiation belt (located in the inner region of Earth's magnetosphere), with the follow-up Explorer 3 later that year definitively proving its existence. Also during 1958, Eugene Parker proposed the idea of the solar wind, with the term 'magnetosphere' being proposed by Thomas Gold in 1959 to explain how the solar wind interacted with the Earth's magnetic field. The later mission of Explorer 12 in 1961 led by the Cahill and Amazeen observation in 1963 of a sudden decrease in magnetic field strength near the noon-time meridian, later was named the magnetopause. By 1983, the International Cometary Explorer observed the magnetotail, or the distant magnetic field.
Structure and behavior
Magnetospheres are dependent on several variables: the type of astronomical object, the nature of sources of plasma and momentum, the period of the object's spin, the nature of the axis about which the object spins, the axis of the magnetic dipole, and the magnitude and direction of the flow of solar wind.
The planetary distance where the magnetosphere can withstand the solar wind pressure is called the Chapman–Ferraro distance. This is usefully modeled by the formula wherein
represents the radius of the planet, represents the magnetic field on the surface of the planet at the equator, and represents the velocity of the solar wind:A magnetosphere is classified as "intrinsic" when Mercury, Earth, Jupiter, Ganymede, Saturn, Uranus, and Neptune, for example, exhibit intrinsic magnetospheres. A magnetosphere is classified as "induced" when , or when the solar wind is not opposed by the object's magnetic field. In this case, the solar wind interacts with the atmosphere or ionosphere of the planet (or surface of the planet, if the planet has no atmosphere). Venus has an induced magnetic field, which means that because Venus appears to have no internal dynamo effect, the only magnetic field present is that formed by the solar wind's wrapping around the physical obstacle of Venus (see also Venus' induced magnetosphere). When , the planet itself and its magnetic field both contribute. It is possible that Mars is of this type.
, or when the primary opposition to the flow of solar wind is the magnetic field of the object.Structure
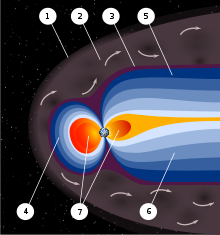
Bow shock

The bow shock forms the outermost layer of the magnetosphere; the boundary between the magnetosphere and the ambient medium. For stars, this is usually the boundary between the stellar wind and interstellar medium; for planets, the speed of the solar wind there decreases as it approaches the magnetopause. Due to interactions with the bow shock, the stellar wind plasma gains a substantial anisotropy, leading to various plasma instabilities upstream and downstream of the bow shock.
Magnetosheath
Main article: MagnetosheathThe magnetosheath is the region of the magnetosphere between the bow shock and the magnetopause. It is formed mainly from shocked solar wind, though it contains a small amount of plasma from the magnetosphere. It is an area exhibiting high particle energy flux, where the direction and magnitude of the magnetic field varies erratically. This is caused by the collection of solar wind gas that has effectively undergone thermalization. It acts as a cushion that transmits the pressure from the flow of the solar wind and the barrier of the magnetic field from the object.
Magnetopause
Main article: MagnetopauseThe magnetopause is the area of the magnetosphere wherein the pressure from the planetary magnetic field is balanced with the pressure from the solar wind. It is the convergence of the shocked solar wind from the magnetosheath with the magnetic field of the object and plasma from the magnetosphere. Because both sides of this convergence contain magnetized plasma, the interactions between them are complex. The structure of the magnetopause depends upon the Mach number and beta of the plasma, as well as the magnetic field. The magnetopause changes size and shape as the pressure from the solar wind fluctuates.
Magnetotail
Opposite the compressed magnetic field is the magnetotail, where the magnetosphere extends far beyond the astronomical object. It contains two lobes, referred to as the northern and southern tail lobes. Magnetic field lines in the northern tail lobe point towards the object while those in the southern tail lobe point away. The tail lobes are almost empty, with few charged particles opposing the flow of the solar wind. The two lobes are separated by a plasma sheet, an area where the magnetic field is weaker, and the density of charged particles is higher.
Earth's magnetosphere
See also: Earth's magnetic field § Magnetosphere, and Van Allen radiation belt Further information: Plasmasphere

Over Earth's equator, the magnetic field lines become almost horizontal, then return to reconnect at high latitudes. However, at high altitudes, the magnetic field is significantly distorted by the solar wind and its solar magnetic field. On the dayside of Earth, the magnetic field is significantly compressed by the solar wind to a distance of approximately 65,000 kilometers (40,000 mi). Earth's bow shock is about 17 kilometers (11 mi) thick and located about 90,000 kilometers (56,000 mi) from Earth. The magnetopause exists at a distance of several hundred kilometers above Earth's surface. Earth's magnetopause has been compared to a sieve because it allows solar wind particles to enter. Kelvin–Helmholtz instabilities occur when large swirls of plasma travel along the edge of the magnetosphere at a different velocity from the magnetosphere, causing the plasma to slip past. This results in magnetic reconnection, and as the magnetic field lines break and reconnect, solar wind particles are able to enter the magnetosphere. On Earth's nightside, the magnetic field extends in the magnetotail, which lengthwise exceeds 6,300,000 kilometers (3,900,000 mi). Earth's magnetotail is the primary source of the polar aurora. Also, NASA scientists have suggested that Earth's magnetotail might cause "dust storms" on the Moon by creating a potential difference between the day side and the night side.
Other objects
Many astronomical objects generate and maintain magnetospheres. In the Solar System this includes the Sun, Mercury, Earth, Jupiter, Saturn, Uranus, Neptune, and Ganymede. The magnetosphere of Jupiter is the largest planetary magnetosphere in the Solar System, extending up to 7,000,000 kilometers (4,300,000 mi) on the dayside and almost to the orbit of Saturn on the nightside. Jupiter's magnetosphere is stronger than Earth's by an order of magnitude, and its magnetic moment is approximately 18,000 times larger. Venus, Mars, and Pluto, on the other hand, have no magnetic field. This may have had significant effects on their geological history. It is theorized that Venus and Mars may have lost their primordial water to photodissociation and the solar wind. A strong magnetosphere greatly slows this process.

Magnetospheres generated by exoplanets are thought to be common, though the first discoveries did not come until the 2010s. In 2014, a magnetic field around HD 209458 b was inferred from the way hydrogen was evaporating from the planet. In 2019, the strength of the surface magnetic fields of 4 hot Jupiters were estimated and ranged between 20 and 120 gauss compared to Jupiter's surface magnetic field of 4.3 gauss. In 2020, a radio emission in the 14-30 MHz band was detected from the Tau Boötis system, likely associated with cyclotron radiation from the poles of Tau Boötis b a signature of a planetary magnetic field. In 2021 a magnetic field generated by HAT-P-11b became the first to be confirmed. The first unconfirmed detection of a magnetic field generated by a terrestrial exoplanet was found in 2023 on YZ Ceti b.
See also
References
- "Magnetospheres". NASA Science. NASA.
- Ratcliffe, John Ashworth (1972). An Introduction to the Ionosphere and Magnetosphere. CUP Archive. ISBN 9780521083416.
- ^ "Ionosphere and magnetosphere". Encyclopædia Britannica. Encyclopædia Britannica, Inc. 2012.
- ^ Van Allen, James Alfred (2004). Origins of Magnetospheric Physics. Iowa City, Iowa USA: University of Iowa Press. ISBN 9780877459217. OCLC 646887856.
- Blanc, M.; Kallenbach, R.; Erkaev, N.V. (2005). "Solar System Magnetospheres". Space Science Reviews. 116 (1–2): 227–298. Bibcode:2005SSRv..116..227B. doi:10.1007/s11214-005-1958-y. S2CID 122318569.
- Sparavigna, A.C.; Marazzato, R. (10 May 2010). "Observing stellar bow shocks". arXiv:1005.1527 .
- Pokhotelov, D.; von Alfthan, S.; Kempf, Y.; Vainio, R.; et al. (17 December 2013). "Ion distributions upstream and downstream of the Earth's bow shock: first results from Vlasiator". Annales Geophysicae. 31 (12): 2207–2212. Bibcode:2013AnGeo..31.2207P. doi:10.5194/angeo-31-2207-2013.
- Paschmann, G.; Schwartz, S.J.; Escoubet, C.P.; Haaland, S., eds. (2005). Outer Magnetospheric Boundaries: Cluster Results (PDF). Space Sciences Series of ISSI. Vol. 118. Bibcode:2005ombc.book.....P. doi:10.1007/1-4020-4582-4. ISBN 978-1-4020-3488-6.
{{cite book}}
:|journal=
ignored (help) - Russell, C.T. (1990). "The Magnetopause". In Russell, C.T.; Priest, E.R.; Lee, L.C. (eds.). Physics of magnetic flux ropes. American Geophysical Union. pp. 439–453. ISBN 9780875900261. Archived from the original on 2 February 1999.
- Stern, David P.; Peredo, Mauricio (20 November 2003). "The Magnetopause". The Exploration of the Earth's Magnetosphere. NASA. Archived from the original on 19 August 2019. Retrieved 19 August 2019.
- ^ "The Tail of the Magnetosphere". NASA. Archived from the original on 7 February 2018. Retrieved 22 December 2012.
- "Cluster reveals Earth's bow shock is remarkably thin". European Space Agency. 16 November 2011.
- "Cluster reveals the reformation of Earth's bow shock". European Space Agency. 11 May 2011.
- "Cluster observes a 'porous' magnetopause". European Space Agency. 24 October 2012.
- http://www.nasa.gov/topics/moonmars/features/magnetotail_080416.html Archived 14 November 2021 at the Wayback Machine NASA, The Moon and the Magnetotail
- ^ "Planetary Shields: Magnetospheres". NASA. Retrieved 5 January 2020.
- Khurana, K. K.; Kivelson, M. G.; et al. (2004). "The configuration of Jupiter's magnetosphere" (PDF). In Bagenal, Fran; Dowling, Timothy E.; McKinnon, William B. (eds.). Jupiter: The Planet, Satellites and Magnetosphere. Cambridge University Press. ISBN 978-0-521-81808-7.
- Russell, C.T. (1993). "Planetary Magnetospheres". Reports on Progress in Physics. 56 (6): 687–732. Bibcode:1993RPPh...56..687R. doi:10.1088/0034-4885/56/6/001. S2CID 250897924.
- NASA (14 September 2016). "X-ray Detection Sheds New Light on Pluto". nasa.gov. Retrieved 3 December 2016.
- Charles Q. Choi (20 November 2014). "Unlocking the Secrets of an Alien World's Magnetic Field". Space.com. Retrieved 17 January 2022.
- Kislyakova, K. G.; Holmstrom, M.; Lammer, H.; Odert, P.; Khodachenko, M. L. (2014). "Magnetic moment and plasma environment of HD 209458b as determined from Ly observations". Science. 346 (6212): 981–984. arXiv:1411.6875. Bibcode:2014Sci...346..981K. doi:10.1126/science.1257829. PMID 25414310. S2CID 206560188.
- Passant Rabie (29 July 2019). "Magnetic Fields of 'Hot Jupiter' Exoplanets Are Much Stronger Than We Thought". Space.com. Retrieved 17 January 2022.
- Cauley, P. Wilson; Shkolnik, Evgenya L.; Llama, Joe; Lanza, Antonino F. (December 2019). "Magnetic field strengths of hot Jupiters from signals of star-planet interactions". Nature Astronomy. 3 (12): 1128–1134. arXiv:1907.09068. Bibcode:2019NatAs...3.1128C. doi:10.1038/s41550-019-0840-x. ISSN 2397-3366. S2CID 198147426.
- Turner, Jake D.; Zarka, Philippe; Grießmeier, Jean-Mathias; Lazio, Joseph; Cecconi, Baptiste; Emilio Enriquez, J.; Girard, Julien N.; Jayawardhana, Ray; Lamy, Laurent; Nichols, Jonathan D.; De Pater, Imke (2021), "The search for radio emission from the exoplanetary systems 55 Cancri, υ Andromedae, and τ Boötis using LOFAR beam-formed observations", Astronomy & Astrophysics, 645: A59, arXiv:2012.07926, Bibcode:2021A&A...645A..59T, doi:10.1051/0004-6361/201937201, S2CID 212883637
- O'Callaghan, Jonathan (7 August 2023). "Exoplanets Could Help Us Learn How Planets Make Magnetism". Quanta Magazine. Retrieved 7 August 2023.
- HAT-P-11 Spectral Energy Distribution Signatures of Strong Magnetization and Metal-poor Atmosphere for a Neptune-Size Exoplanet, Ben-Jaffel et al. 2021
- Pineda, J. Sebastian; Villadsen, Jackie (April 2023). "Coherent radio bursts from known M-dwarf planet host YZ Ceti". Nature Astronomy. 7 (5): 569–578. arXiv:2304.00031. Bibcode:2023NatAs...7..569P. doi:10.1038/s41550-023-01914-0.
- Trigilio, Corrado; Biswas, Ayan; et al. (May 2023). "Star-Planet Interaction at radio wavelengths in YZ Ceti: Inferring planetary magnetic field". arXiv:2305.00809 .
- "A magnetic field on a nearby Earth-sized exoplanet?". earthsky.org. 10 April 2023. Retrieved 7 August 2023.
- O'Callaghan, Jonathan (7 August 2023). "Exoplanets Could Help Us Learn How Planets Make Magnetism". Quanta Magazine.
Magnetospherics | |
---|---|
Submagnetosphere | |
Earth's magnetosphere | |
Solar wind | |
Satellites |
|
Research projects | |
Other magnetospheres | |
Related topics |
... in space | ||||||
---|---|---|---|---|---|---|
Biology |
| ![]() | ||||
Environment | ||||||
Society | ||||||
Technology |
| |||||
![]() |
Categories: