Revision as of 06:19, 9 August 2014 editShravya77 (talk | contribs)1 editmNo edit summaryTag: gettingstarted edit← Previous edit | Latest revision as of 12:29, 29 January 2024 edit undoTc14Hd (talk | contribs)Extended confirmed users1,367 editsNo edit summaryTags: Mobile edit Mobile app edit Android app edit | ||
(36 intermediate revisions by 25 users not shown) | |||
Line 1: | Line 1: | ||
{{Short description|Chemical bond involving ten bonding electrons}} | |||
<sub>2</sub>]] | |||
A '''quintuple bond''' in ] is an unusual type of ], first reported in 2005 for a |
<sub>2</sub>]] | ||
A '''quintuple bond''' in ] is an unusual type of ], first reported in 2005 for a ] compound. ]s, ]s, and ]s are commonplace in chemistry. ]s are rarer and are currently known only among the transition metals, especially for ], ], ], and ], e.g. <sup>4−</sup> and <sup>2−</sup>. In a quintuple bond, ten electrons participate in bonding between the two metal centers, allocated as σ<sup>2</sup>π<sup>4</sup>δ<sup>4</sup>. | |||
In some cases of high-order bonds between metal atoms, the metal-metal bonding is facilitated by ligands that link the two metal centers and reduce the interatomic distance. By contrast, the chromium dimer with quintuple bonding is stabilized by bulky ] (2,6-phenyl) ]s. The species is stable up to 200 °C.<ref> |
In some cases of high-order bonds between metal atoms, the metal-metal bonding is facilitated by ligands that link the two metal centers and reduce the interatomic distance. By contrast, the chromium dimer with quintuple bonding is stabilized by a bulky ] (2,6-phenyl) ]s. The species is stable up to 200 °C.<ref>{{cite journal|url=http://pubs.acs.org/cen/news/83/i39/8339notw1.html|title=Quintuple Bond Makes Its Debut: First stable molecule with fivefold metal–metal bonding is synthesized|first=Steve|last=Ritter|journal=]|date=26 September 2005|volume=83|issue=39}}</ref><ref>{{cite journal|title=Synthesis of a Stable Compound with Fivefold Bonding Between Two Chromium(I) Centers|first1=Tailuan|last1=Nguyen|first2=Andrew D.|last2=Sutton|first3=Marcin|last3=Brynda|first4=James C.|last4=Fettinger|first5=Gary J.|last5=Long|author6-link=Philip Power|first6=Philip P.|last6=Power|date=2005|doi=10.1126/science.1116789|journal=]|volume=310|issue=5749|pages=844–847|pmid=16179432|bibcode=2005Sci...310..844N|s2cid=42853922|doi-access=free}}</ref> The chromium–chromium quintuple bond has been analyzed with multireference ''ab initio'' and ] methods,<ref>{{cite journal|title=Quantum Chemical Study of the Quintuple Bond between Two Chromium Centers in : ''trans''-Bent versus Linear Geometry|first1=Marcin|last1=Brynda|first2=Laura|last2=Gagliardi|first3=Per-Olof|last3=Widmark|first4=Philip P.|last4=Power|first5=Björn O.|last5=Roos|journal=]|volume=45|issue=23|pages=3804–3807|date=2006|doi=10.1002/anie.200600110|pmid = 16671122|url = http://archive-ouverte.unige.ch/unige:3645}}{{open access}}</ref> which were also used to elucidate the role of the terphenyl ligand, in which the flanking aryls were shown to interact very weakly with the chromium atoms, causing only a small weakening of the quintuple bond.<ref>{{cite journal|title=Large Differences in Secondary Metal−Arene Interactions in the Transition-Metal Dimers ArMMAr (Ar = Terphenyl; M = Cr, Fe, or Co): Implications for Cr−Cr Quintuple Bonding|first1=Giovanni|last1=La Macchia|first2=Laura|last2=Gagliardi|first3=Philip P.|last3=Power|first4=Marcin|last4=Brynda|journal=]|date=2008|volume=130|issue=15|pages=5104–5114|doi=10.1021/ja0771890|pmid=18335988|s2cid=207046428}}</ref> A 2007 theoretical study identified two global minima for quintuple bonded RMMR compounds: a ''trans''-bent ] and surprisingly another ''trans''-bent geometry with the R substituent in a bridging position.<ref>{{cite journal|title=The Many Ways To Have a Quintuple Bond|first1=Gabriel|last1=Merino|first2=Kelling J.|last2=Donald|first3=Jason S.|last3=D'Acchioli|author4-link=Roald Hoffmann|first4=Roald|last4=Hoffmann|journal=]|date=2007|volume=129|issue=49|pages=15295–15302|doi=10.1021/ja075454b|pmid=18004851|s2cid=18838267}}</ref> | ||
In 2005, a quintuple bond was postulated to exist in the hypothetical ] molecule U<sub>2</sub> based on ].<ref> |
In 2005, a quintuple bond was postulated to exist in the hypothetical ] molecule U<sub>2</sub> based on ].<ref>{{cite journal|title=Quantum chemical calculations show that the uranium molecule U<sub>2</sub> has a quintuple bond|first1=Laura|last1=Gagliardi|first2=Björn O.|last2=Roos|journal=]|volume=433|issue=7028|pages=848–851|date=24 February 2005|doi=10.1038/nature03249|pmid=15729337|bibcode=2005Natur.433..848G|s2cid=421380 |url=https://archive-ouverte.unige.ch/unige:3652/ATTACHMENT01|author1-link=Laura Gagliardi }}</ref><ref>{{cite news|url=http://physicsweb.org/articles/news/9/2/13/1#050213|title=New look for chemical bonds|first=Belle|last=Dumé|date=23 February 2005|website=PhysicsWeb}}</ref> Diuranium compounds are rare, but do exist; for example, the {{chem|U|2|Cl|8|2−}} anion. | ||
In 2007 the shortest |
In 2007 the shortest-ever metal–metal bond (180.28 pm) was reported to exist also in a compound containing a quintuple chromium-chromium bond with diazadiene bridging ligands.<ref>{{cite journal|title=The Shortest Metal–Metal Bond Yet: Molecular and Electronic Structure of a Dinuclear Chromium Diazadiene Complex|first1=Kevin A.|last1=Kreisel|first2=Glenn P. A.|last2=Yap|first3=Olga|last3=Dmitrenko|first4=Clark R.|last4=Landis|first5=Klaus H.|last5=Theopold|date=2007|journal=]|volume=129|issue=46|pages=14162–14163|type=Communication|doi=10.1021/ja076356t|pmid=17967028}}</ref> Other metal–metal quintuple bond containing complexes that have been reported include quintuply bonded dichromium with (2,4,6-trimethylphenyl)amine bridging ligands<ref>{{cite journal|title=Metal–Metal Distances at the Limit: A Coordination Compound with an Ultrashort Chromium–Chromium Bond|first1=Awal|last1=Noor|first2=Frank R.|last2=Wagner|first3=Rhett|last3=Kempe|journal=]|date=2008|volume=47|issue=38|pages=7246–7249|doi=10.1002/anie.200801160|pmid=18698657|s2cid=30480347}}</ref> and a dichromium complex with ] bridging ligands.<ref>{{cite journal|title=Remarkably Short Metal–Metal Bonds: A Lantern-Type Quintuply Bonded Dichromium(I) Complex|first1=Yi-Chou|last1=Tsai|first2=Chia-Wei|last2=Hsu|first3=Jen-Shiang K.|last3=Yu|first4=Gene-Hsiang|last4=Lee|first5=Yu|last5=Wang|first6=Ting-Shen|last6=Kuo|journal=]|date=2008|volume=47|issue=38|pages=7250–7253|doi=10.1002/anie.200801286|pmid=18683844|s2cid=5510753}}</ref> | ||
Synthesis of quintuple bonds is usually achieved through reduction of a dimetal species using ]. This adds ]s to the metal centers, giving them the needed number of electrons to participate in quintuple bonding. Below is a figure of a typical quintuple bond synthesis. | Synthesis of quintuple bonds is usually achieved through reduction of a dimetal species using ]. This adds ]s to the metal centers, giving them the needed number of electrons to participate in quintuple bonding. Below is a figure of a typical quintuple bond synthesis. | ||
] | :] | ||
==Dimolybdenum quintuple bonds== | ==Dimolybdenum quintuple bonds== | ||
⚫ | In 2009 a dimolybdenum compound with a quintuple bond and two di] bridging ligands was reported with a Mo–Mo bond length of 202 pm.<ref>{{cite journal|title=Journey from Mo–Mo Quadruple Bonds to Quintuple Bonds|first1=Yi-Chou|last1=Tsai|first2=Hong-Zhang|last2=Chen|first3=Chie-Chieh|last3=Chang|first4=Jen-Shiang K.|last4=Yu|first5=Gene-Hsiang|last5=Lee|first6=Yu|last6=Wang|first7=Ting-Shen|last7=Kuo|journal=J. Am. Chem. Soc.|date=2009|volume=131|issue=35|pages=12534–12535|doi=10.1021/ja905035f|pmid=19685872|s2cid=207144833}}</ref> The compound was synthesised starting from ] (which already contains a Mo<sub>2</sub> quadruple bond) and a lithium amidinate, followed by reduction with potassium graphite: | ||
⚫ | :] | ||
⚫ | In 2009 a dimolybdenum compound with a quintuple bond and two |
||
⚫ | ] | ||
==Bonding== | ==Bonding== | ||
⚫ | As stated above metal-metal quintuple bonds have a σ<sup>2</sup>π<sup>4</sup>δ<sup>4</sup> configuration. Among the five bonds present between the metal centers, one is a ], two are ]s, and two are ]s. The σ-bond is the result of mixing between the d<sub>''z''<sup>2</sup></sub> orbital on each metal center. The first π-bond comes from mixing of the d<sub>''yz''</sub> orbitals from each metal while the other π-bond comes from the d<sub>''xz''</sub> orbitals on each metal mixing. Finally the δ-bonds come from mixing of the d<sub>''xy''</sub> orbitals as well as mixing between the d<sub>''x''<sup>2</sup>−''y''<sup>2</sup></sub> orbitals from each metal. | ||
⚫ | Molecular orbital calculations have elucidated the relative energies of the orbitals created by these bonding interactions. As shown in the figure below, the lowest energy orbitals are the π bonding orbitals followed by the σ bonding orbital. The next highest are the δ bonding orbitals which represent the ]. Because the 10 valence electrons of the metals are used to fill these first 5 orbitals, the next highest orbital becomes the ] which is the δ* ] orbital. Though the π and δ orbitals are represented as being ], they in fact are not. This is because the model shown here is a simplification and that hybridization of s, p, and d orbitals is believed to take place, causing a change in the orbital energy levels.{{Citation needed|reason=A reference to the molecular orbital calculation that has π < σ < δ is needed. The expected molecular orbital ordering would be σ < π < δ.|date=December 2019}} | ||
⚫ | As stated above metal-metal quintuple bonds have a σ<sup>2</sup>π<sup>4</sup>δ<sup>4</sup> configuration. Among the five bonds present between the metal centers, one is a ], two are ]s, and two are ]s. |
||
⚫ | ] | ||
⚫ | Molecular orbital calculations have elucidated the relative energies of the orbitals created by these bonding interactions. As shown in the figure below, the lowest energy orbitals are the |
||
⚫ | ==Ligand role in metal–metal quintuple bond length== | ||
⚫ | ] | ||
⚫ | Quintuple bond lengths are heavily dependent on the ligands bound to the metal centers. Nearly all complexes containing a metal–metal quintuple bond have ] bridging ligands, and even those that do not, such as the terphenyl complex mentioned earlier, have some bridging characteristic to it through metal–]-carbon interactions. | ||
⚫ | The bidentate ligand can act as a sort of tweezer in that in order for ] to occur the metal atoms must move closer together, thereby shortening the quintuple bond length. The two ways in which to obtain shorter metal–metal distances is to either reduce the distance between the chelating atoms in the ligand by changing the structure, or by using ] to force a conformational change in the ligand that bends the molecule in a way that forces the chelating atoms closer together. An example of the latter is shown below: | ||
⚫ | ==Ligand role in |
||
⚫ | :] | ||
⚫ | Quintuple bond lengths |
||
⚫ | The bidentate ligand can act as a sort of tweezer in that in order for ] to occur the metal atoms must move closer together, thereby shortening the quintuple bond length. The two ways in which to obtain shorter |
||
⚫ | The above example shows the ligand used in the dimolybdenum complex shown earlier. When the carbon between the two nitrogens in the ligand has a hydrogen bound to it, the steric repulsion is small. However, when the hydrogen is replaced with a much more bulky phenyl ring the steric repulsion increases dramatically and the ligand "bows" which causes a change in the orientation of the lone pairs of electrons on the nitrogen atoms. These lone pairs are what is responsible for forming bonds with the metal centers so forcing them to move closer together also forces the metal centers to be positioned closer together. Thus, decreasing the length of the quintuple bond. In the case where this ligand is bound to quintuply bonded dimolybdenum the quintuple bond length goes from 201.87 pm to 201.57 pm when the hydrogen in replaced with a phenyl group. Similar results have also been demonstrated in dichromium quintuple bond complexes as well.<ref>{{cite journal|title=Quintuply-Bonded Dichromium(I) Complexes Featuring Metal–Metal Bond Lengths of 1.74 Å|first1=Chai-Wei|last1=Hsu|first2=Jen-Shiang K.|last2=Yu|first3=Chun-Hsu|last3=Yen|first4=Gene-Hsiang|last4=Lee|first5=Yu|last5=Wang|first6=Yi-Chou|last6=Tsa|journal=Angew. Chem. Int. Ed.|date=2008|volume=47|issue=51|pages=9933–9936|doi=10.1002/anie.200803859|pmid=19016281|s2cid=46033904}}</ref> | ||
⚫ | ] | ||
==Research trends== | |||
⚫ | The above example shows the ligand used in the dimolybdenum complex shown |
||
Efforts continue to prepare shorter quintuple bonds.<ref>{{cite journal|title=Carboalumination of a chromium–chromium quintuple bond|first1=Awal|last1=Noor|first2=Germund|last2=Glatz|first3=Robert|last3=Muller|first4=Martin|last4=Kaupp|first5=Serhiy|last5=Demeshko|first6=Rhett|last6=Kempe|journal=]|date=2009|volume=1|issue=4|pages=322–325|doi=10.1038/NCHEM.255|pmid=21500603|bibcode=2009NatCh...1..322N}}</ref><ref>{{cite journal|title=Reactions of Ar′CrCrAr′ with N<sub>2</sub>O or N<sub>3</sub>(1-Ad): complete cleavage of the Cr–Cr quintuple bond interaction|first1=Chengbao|last1=Ni|first2=Bobby D.|last2=Ellis|first3=Gary J.|last3=Long|first4=Philip P.|last4=Power|journal=]|date=2009|volume=2009|issue=17|pages=2332–2334|doi=10.1039/b901494b|pmid=19377676}}</ref> | |||
Quintuple-bonded dichromium complexes appear to act like magnesium to produce ]s.<ref>{{cite journal|url=https://pubs.acs.org/doi/abs/10.1021/om501230g|title=Low-Valent Aminopyridinato Chromium Methyl Complexes via Reductive Alkylation and via Oxidative Addition of Iodomethane by a Cr–Cr Quintuple Bond|last1=Noor|first1=Awal|last2=Schwarz|first2=Stefan|last3=Kempe|first3=Rhett|journal=Organometallics|date=9 Feb 2015|volume=34|issue=11|pages=2122–2125|doi=10.1021/om501230g}}</ref> | |||
==Future prospects== | |||
Research into quintuple bonds is still in its infancy and as a result is more of an interesting phenomenon that has no practical applications yet. These quintuple bond-containing ] do not seem to lend themselves for use as ] due to their high reactivity. Current research is centered mostly on making shorter quintuple bonds, though new reactions have been reported<ref>''Carboalumination of a chromium-chromium quintuple bond'' Awal Noor, Germund Glatz, Robert Muller, Martin Kaupp, Serhiy Demeshko, and Rhett Kempe ] Published online 22 June 2009 {{DOI|10.1038/NCHEM.255}}</ref><ref>''Reactions of Ar'CrCrAr' with N<sub>2</sub>O or N<sub>3</sub>(1-Ad): complete cleavage of the Cr-Cr quintuple bond interaction'' Chengbao Ni, Bobby D. Ellis, Gary J. Long, and Phillip P. Power ] 2009 {{DOI|10.1039/b901494b}}</ref> that have led to rarely seen metal-ligand interactions. This suggests metal-metal quintuply bonded complexes could be used as precursors to make previously unattainable compounds. | |||
== References == | == References == | ||
⚫ | {{reflist|30em}} | ||
⚫ | {{Wikinews|Delaware scientists create shortest ever metal to metal bond}} | ||
⚫ | {{reflist}} | ||
== See also == | == See also == | ||
⚫ | {{Wikinews|Delaware scientists create shortest ever metal to metal bond}} | ||
*] | |||
{{Chemical bonding theory}} | |||
{{chemical bonds}} | |||
] | ] | ||
] |
Latest revision as of 12:29, 29 January 2024
Chemical bond involving ten bonding electrons
A quintuple bond in chemistry is an unusual type of chemical bond, first reported in 2005 for a dichromium compound. Single bonds, double bonds, and triple bonds are commonplace in chemistry. Quadruple bonds are rarer and are currently known only among the transition metals, especially for Cr, Mo, W, and Re, e.g. and . In a quintuple bond, ten electrons participate in bonding between the two metal centers, allocated as σπδ.
In some cases of high-order bonds between metal atoms, the metal-metal bonding is facilitated by ligands that link the two metal centers and reduce the interatomic distance. By contrast, the chromium dimer with quintuple bonding is stabilized by a bulky terphenyl (2,6-phenyl) ligands. The species is stable up to 200 °C. The chromium–chromium quintuple bond has been analyzed with multireference ab initio and DFT methods, which were also used to elucidate the role of the terphenyl ligand, in which the flanking aryls were shown to interact very weakly with the chromium atoms, causing only a small weakening of the quintuple bond. A 2007 theoretical study identified two global minima for quintuple bonded RMMR compounds: a trans-bent molecular geometry and surprisingly another trans-bent geometry with the R substituent in a bridging position.
In 2005, a quintuple bond was postulated to exist in the hypothetical uranium molecule U2 based on computational chemistry. Diuranium compounds are rare, but do exist; for example, the U
2Cl
8 anion.
In 2007 the shortest-ever metal–metal bond (180.28 pm) was reported to exist also in a compound containing a quintuple chromium-chromium bond with diazadiene bridging ligands. Other metal–metal quintuple bond containing complexes that have been reported include quintuply bonded dichromium with (2,4,6-trimethylphenyl)amine bridging ligands and a dichromium complex with amidinate bridging ligands.
Synthesis of quintuple bonds is usually achieved through reduction of a dimetal species using potassium graphite. This adds valence electrons to the metal centers, giving them the needed number of electrons to participate in quintuple bonding. Below is a figure of a typical quintuple bond synthesis.
Dimolybdenum quintuple bonds
In 2009 a dimolybdenum compound with a quintuple bond and two diamido bridging ligands was reported with a Mo–Mo bond length of 202 pm. The compound was synthesised starting from potassium octachlorodimolybdate (which already contains a Mo2 quadruple bond) and a lithium amidinate, followed by reduction with potassium graphite:
Bonding
As stated above metal-metal quintuple bonds have a σπδ configuration. Among the five bonds present between the metal centers, one is a sigma bond, two are pi bonds, and two are delta bonds. The σ-bond is the result of mixing between the dz orbital on each metal center. The first π-bond comes from mixing of the dyz orbitals from each metal while the other π-bond comes from the dxz orbitals on each metal mixing. Finally the δ-bonds come from mixing of the dxy orbitals as well as mixing between the dx−y orbitals from each metal.
Molecular orbital calculations have elucidated the relative energies of the orbitals created by these bonding interactions. As shown in the figure below, the lowest energy orbitals are the π bonding orbitals followed by the σ bonding orbital. The next highest are the δ bonding orbitals which represent the HOMO. Because the 10 valence electrons of the metals are used to fill these first 5 orbitals, the next highest orbital becomes the LUMO which is the δ* antibonding orbital. Though the π and δ orbitals are represented as being degenerate, they in fact are not. This is because the model shown here is a simplification and that hybridization of s, p, and d orbitals is believed to take place, causing a change in the orbital energy levels.
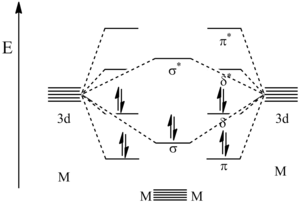
Ligand role in metal–metal quintuple bond length
Quintuple bond lengths are heavily dependent on the ligands bound to the metal centers. Nearly all complexes containing a metal–metal quintuple bond have bidentate bridging ligands, and even those that do not, such as the terphenyl complex mentioned earlier, have some bridging characteristic to it through metal–ipso-carbon interactions.
The bidentate ligand can act as a sort of tweezer in that in order for chelation to occur the metal atoms must move closer together, thereby shortening the quintuple bond length. The two ways in which to obtain shorter metal–metal distances is to either reduce the distance between the chelating atoms in the ligand by changing the structure, or by using steric effects to force a conformational change in the ligand that bends the molecule in a way that forces the chelating atoms closer together. An example of the latter is shown below:
The above example shows the ligand used in the dimolybdenum complex shown earlier. When the carbon between the two nitrogens in the ligand has a hydrogen bound to it, the steric repulsion is small. However, when the hydrogen is replaced with a much more bulky phenyl ring the steric repulsion increases dramatically and the ligand "bows" which causes a change in the orientation of the lone pairs of electrons on the nitrogen atoms. These lone pairs are what is responsible for forming bonds with the metal centers so forcing them to move closer together also forces the metal centers to be positioned closer together. Thus, decreasing the length of the quintuple bond. In the case where this ligand is bound to quintuply bonded dimolybdenum the quintuple bond length goes from 201.87 pm to 201.57 pm when the hydrogen in replaced with a phenyl group. Similar results have also been demonstrated in dichromium quintuple bond complexes as well.
Research trends
Efforts continue to prepare shorter quintuple bonds.
Quintuple-bonded dichromium complexes appear to act like magnesium to produce Grignard reagents.
References
- Ritter, Steve (26 September 2005). "Quintuple Bond Makes Its Debut: First stable molecule with fivefold metal–metal bonding is synthesized". Chemical & Engineering News. 83 (39).
- Nguyen, Tailuan; Sutton, Andrew D.; Brynda, Marcin; Fettinger, James C.; Long, Gary J.; Power, Philip P. (2005). "Synthesis of a Stable Compound with Fivefold Bonding Between Two Chromium(I) Centers". Science. 310 (5749): 844–847. Bibcode:2005Sci...310..844N. doi:10.1126/science.1116789. PMID 16179432. S2CID 42853922.
- Brynda, Marcin; Gagliardi, Laura; Widmark, Per-Olof; Power, Philip P.; Roos, Björn O. (2006). "Quantum Chemical Study of the Quintuple Bond between Two Chromium Centers in [PhCrCrPh]: trans-Bent versus Linear Geometry". Angew. Chem. Int. Ed. 45 (23): 3804–3807. doi:10.1002/anie.200600110. PMID 16671122.
- La Macchia, Giovanni; Gagliardi, Laura; Power, Philip P.; Brynda, Marcin (2008). "Large Differences in Secondary Metal−Arene Interactions in the Transition-Metal Dimers ArMMAr (Ar = Terphenyl; M = Cr, Fe, or Co): Implications for Cr−Cr Quintuple Bonding". J. Am. Chem. Soc. 130 (15): 5104–5114. doi:10.1021/ja0771890. PMID 18335988. S2CID 207046428.
- Merino, Gabriel; Donald, Kelling J.; D'Acchioli, Jason S.; Hoffmann, Roald (2007). "The Many Ways To Have a Quintuple Bond". J. Am. Chem. Soc. 129 (49): 15295–15302. doi:10.1021/ja075454b. PMID 18004851. S2CID 18838267.
- Gagliardi, Laura; Roos, Björn O. (24 February 2005). "Quantum chemical calculations show that the uranium molecule U2 has a quintuple bond". Nature. 433 (7028): 848–851. Bibcode:2005Natur.433..848G. doi:10.1038/nature03249. PMID 15729337. S2CID 421380.
- Dumé, Belle (23 February 2005). "New look for chemical bonds". PhysicsWeb.
- Kreisel, Kevin A.; Yap, Glenn P. A.; Dmitrenko, Olga; Landis, Clark R.; Theopold, Klaus H. (2007). "The Shortest Metal–Metal Bond Yet: Molecular and Electronic Structure of a Dinuclear Chromium Diazadiene Complex". J. Am. Chem. Soc. (Communication). 129 (46): 14162–14163. doi:10.1021/ja076356t. PMID 17967028.
- Noor, Awal; Wagner, Frank R.; Kempe, Rhett (2008). "Metal–Metal Distances at the Limit: A Coordination Compound with an Ultrashort Chromium–Chromium Bond". Angew. Chem. Int. Ed. 47 (38): 7246–7249. doi:10.1002/anie.200801160. PMID 18698657. S2CID 30480347.
- Tsai, Yi-Chou; Hsu, Chia-Wei; Yu, Jen-Shiang K.; Lee, Gene-Hsiang; Wang, Yu; Kuo, Ting-Shen (2008). "Remarkably Short Metal–Metal Bonds: A Lantern-Type Quintuply Bonded Dichromium(I) Complex". Angew. Chem. Int. Ed. 47 (38): 7250–7253. doi:10.1002/anie.200801286. PMID 18683844. S2CID 5510753.
- Tsai, Yi-Chou; Chen, Hong-Zhang; Chang, Chie-Chieh; Yu, Jen-Shiang K.; Lee, Gene-Hsiang; Wang, Yu; Kuo, Ting-Shen (2009). "Journey from Mo–Mo Quadruple Bonds to Quintuple Bonds". J. Am. Chem. Soc. 131 (35): 12534–12535. doi:10.1021/ja905035f. PMID 19685872. S2CID 207144833.
- Hsu, Chai-Wei; Yu, Jen-Shiang K.; Yen, Chun-Hsu; Lee, Gene-Hsiang; Wang, Yu; Tsa, Yi-Chou (2008). "Quintuply-Bonded Dichromium(I) Complexes Featuring Metal–Metal Bond Lengths of 1.74 Å". Angew. Chem. Int. Ed. 47 (51): 9933–9936. doi:10.1002/anie.200803859. PMID 19016281. S2CID 46033904.
- Noor, Awal; Glatz, Germund; Muller, Robert; Kaupp, Martin; Demeshko, Serhiy; Kempe, Rhett (2009). "Carboalumination of a chromium–chromium quintuple bond". Nature Chemistry. 1 (4): 322–325. Bibcode:2009NatCh...1..322N. doi:10.1038/NCHEM.255. PMID 21500603.
- Ni, Chengbao; Ellis, Bobby D.; Long, Gary J.; Power, Philip P. (2009). "Reactions of Ar′CrCrAr′ with N2O or N3(1-Ad): complete cleavage of the Cr–Cr quintuple bond interaction". Chemical Communications. 2009 (17): 2332–2334. doi:10.1039/b901494b. PMID 19377676.
- Noor, Awal; Schwarz, Stefan; Kempe, Rhett (9 Feb 2015). "Low-Valent Aminopyridinato Chromium Methyl Complexes via Reductive Alkylation and via Oxidative Addition of Iodomethane by a Cr–Cr Quintuple Bond". Organometallics. 34 (11): 2122–2125. doi:10.1021/om501230g.
See also
Chemical bonding theory | |||||||
---|---|---|---|---|---|---|---|
Types of bonds |
| ||||||
Valence bond theory |
| ||||||
Molecular orbital theory |
|