Revision as of 18:59, 13 October 2006 view sourceAthaenara (talk | contribs)Extended confirmed users54,866 edits →Fire suppression← Previous edit | Revision as of 19:01, 13 October 2006 view source Athaenara (talk | contribs)Extended confirmed users54,866 edits →Reasons why fire suppression may not have affected the fire cycleNext edit → | ||
Line 68: | Line 68: | ||
Several people (Weir et al. 1995, Johnson et al. 1995, 1998) have explored reasons why fire suppression may not have affected the fire cycle. In general, they feel that in closed-canopied forests, like the boreal, as little as 3% of the lightning caused fires account for up to 95% of the area burned (Stocks & Street 1993, Johnson & Wowchuck 1993). Most fires remain small, but a few occur under conditions that allow them to increase rapidly in size. It is this small proportion of large lightning caused fires which has the most influence on the area burned and the fire cycle. | Several people (Weir et al. 1995, Johnson et al. 1995, 1998) have explored reasons why fire suppression may not have affected the fire cycle. In general, they feel that in closed-canopied forests, like the boreal, as little as 3% of the lightning caused fires account for up to 95% of the area burned (Stocks & Street 1993, Johnson & Wowchuck 1993). Most fires remain small, but a few occur under conditions that allow them to increase rapidly in size. It is this small proportion of large lightning caused fires which has the most influence on the area burned and the fire cycle. | ||
In years with a large area burned, fires in these closed-canopied forests characteristically have high intensities, high rates of spread and high duff consumption. In these years, extreme fire behaviour is preceded by a persistent anomalous high pressure system which produces prolonged periods of above normal temperatures and below normal precipitation (Newark 1975, Harrington & Flannigan 1987), and leads to the severe drying of both medium and heavy fuels. Under these extreme conditions, fire behaviour exhibits little difference between aspect, elevation and vegetation type (Anderson 1968, Alexander et al. 1983, Nimchuck 1983, Janz & Nimchuck 1983, Street 1985, Flannigan & Harrington 1988, Fryer & Johnson 1988). In years with only a small area burned, differences in aspect, slope, elevation and vegetation composition can have a significant effect on the fire behaviour (Alexander & McAlpine 1987, Johnson et al 1998), however, the area burned in these years is insignificant. | In years with a large area burned, fires in these closed-canopied forests characteristically have high intensities, high rates of spread and high ] consumption. In these years, extreme fire behaviour is preceded by a persistent anomalous high pressure system which produces prolonged periods of above normal temperatures and below normal precipitation (Newark 1975, Harrington & Flannigan 1987), and leads to the severe drying of both medium and heavy fuels. Under these extreme conditions, fire behaviour exhibits little difference between aspect, elevation and vegetation type (Anderson 1968, Alexander et al. 1983, Nimchuck 1983, Janz & Nimchuck 1983, Street 1985, Flannigan & Harrington 1988, Fryer & Johnson 1988). In years with only a small area burned, differences in aspect, slope, elevation and vegetation composition can have a significant effect on the fire behaviour (Alexander & McAlpine 1987, Johnson et al 1998), however, the area burned in these years is insignificant. | ||
The extreme fire behaviour associated with persistent high pressure systems results in large areas burned. It has been argued that during these years, it is unlikely that fire suppression can significantly influence the total area burned because under these conditions fire management agencies are quickly overwhelmed (Weir et al. 1995). | The extreme fire behaviour associated with persistent high pressure systems results in large areas burned. It has been argued that during these years, it is unlikely that fire suppression can significantly influence the total area burned because under these conditions fire management agencies are quickly overwhelmed (Weir et al. 1995). |
Revision as of 19:01, 13 October 2006
For other uses, see Taiga (disambiguation).
Taiga (IPA pronunciation: /'taɪgə/ or /taɪ 'ga/, from Mongolian) is a biome characterized by coniferous forests. Covering most of inland Alaska, Canada, Sweden, Finland, northern Kazakhstan and Russia (especially Siberia), as well as parts of the extreme northern continental United States, the taiga is the world's largest terrestrial biome and a major source of oxygen. In Canada, boreal forest is the term used to refer to the southern part of this biome, while "taiga" is used to describe the more barren northern areas south of the Arctic tree-line.
Since North America and Eurasia were recently connected by the Bering land bridge, a number of animal and plant species (more animals than plants) were able to colonise both continents and are distributed throughout the taiga biome. Others differ regionally, typically with each genus having several distinct species, each occupying different regions of the taiga.
Climate and geography

The taiga biome has a harsh continental climate with a very large temperature range between summer and winter, classified as "Dfc" or "Dfb" in the Köppen climate classification scheme. Aside from the tundra and permanent ice caps, it is the coldest biome on Earth. High latitudes mean that, for much of the year the sun does not rise far above the horizon; winters last at least 6 months, with average temperatures below freezing. Temperatures vary from -50 °C to 30 °C throughout the whole year, with 8 or more months of temperatures averaging below 10 °C. The summers, while short, are generally warm and humid. In general, taiga grows north to the 10 °C July isotherm, occasionally to the 9 °C July isotherm (Arno & Hammerly 1984, Arno et al. 1995). The southern limit is more variable, depending on rainfall; taiga may be replaced by open steppe woodland south of the 15 °C July isotherm where rainfall is very low, but more typically extends south to the 18 °C July isotherm, and locally where rainfall is higher (notably in eastern Siberia and adjacent northern Manchuria) south to the 20 °C July isotherm. In these warmer areas, the taiga has higher species diversity with more warmth-loving species such as Korean Pine, Jezo Spruce and Manchurian Fir, and merges gradually into mixed temperate forest, or more locally (on the Pacific Ocean coasts of North America and Asia) into coniferous temperate rainforests.
The taiga experiences relatively low precipitation throughout the year (200-750 mm annually), primarily as rain during the summer months, but also as fog and snow; as evaporation is also low for most of the year, precipitation exceeds evaporation and is sufficient for dense vegetation growth. Snow may remain on the ground for as long as nine months in the northernmost extensions of the taiga (Sayre, 16).
Much of the area currently classified as taiga was recently glaciated. As the glaciers receded, they left depressions in the topography that have since filled with water, creating lakes and bogs (especially muskeg soil), found throughout the taiga.
Soils
Taiga soil tends to be young and nutrient-poor; it lacks the deep, organically-enriched profile present in temperate deciduous forests (Sayre, 19). The thinness of the soil is due largely to the cold; it hinders the development of soil, as well as the ease with which plants can use its nutrients (Sayre, 19). Fallen leaves and moss can remain on the forest floor for a long time in the cool, moist climate, which limits their organic contribution to the soil; acids from evergreen needles further leach the soil, creating spodosol (Sayre, 19-20). Since the soil is acidic, the forest floor has only lichens and some mosses growing on it.
Flora
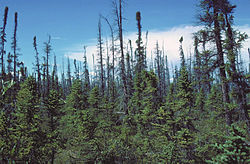
There are two major types of taiga, closed forest, consisting of many closely-spaced trees with mossy ground cover, and lichen woodland, with trees that are farther-spaced and lichen ground cover; the latter is more common in the northernmost taiga (Sayre, 12-3).
The forests of the taiga are largely coniferous, dominated by larch, spruce, fir, and pine. Evergreen species in the taiga (spruce, fir, and pine) have a number of adaptations specifically for survival in harsh taiga winters, though larch, the most cold-tolerant of all trees, is deciduous. Taiga trees tend to have shallow roots to take advantage of the thin soils, while many of them seasonally alter their biochemistry to make them more resistant to freezing, called "hardening" (Sayre, 23). The narrow conical shape of northern conifers, and their downward-drooping limbs, also help them shed snow (Sayre, 23).
Because the sun is low in the horizon for most of the year, it is difficult for plants to generate energy from photosynthesis. Pine and spruce do not lose their leaves seasonally and are able to photosynthesize with their older leaves in late winter and spring when light is good but temperatures are still too low for new growth to commence. The adaptation of evergreen needles limits the water lost due to transpiration and their dark green color increases their absorption of sunlight. Although precipitation is not a limiting factor, the ground freezes during the winter months and plant roots are unable to absorb water, so desiccation can be a severe problem in late winter for evergreens.
Although the taiga is dominated by coniferous forests, some broadleaf trees also occur, notably birch, aspen, willow, and rowan. Many smaller herbaceous plants grow closer to the ground. Periodic stand-replacing wildfires (with return times of between 20-200 years) clear out the tree canopies, allowing sunlight to invigorate new growth on the forest floor. For some species, wildfires are a necessary part of the life cycle in the taiga; some, e.g. Jack Pine have cones which only open to release their seed after a fire, dispersing their seeds onto the newly cleared ground. Grasses grow wherever they can find a patch of sun, and mosses and lichens thrive on the damp ground and on the sides of tree trunks. In comparison with other biomes, however, the taiga has a low biological diversity.
Fauna
The taiga is home to a number of large herbivorous mammals and smaller rodents. These animals have also adapted to survive the harsh climate. Some of the larger mammals, such as bears, eat during the summer in order to gain weight and then go into hibernation during the winter. Other animals have adapted layers of fur or feathers to insulate them from the cold.
Due to the climate, carnivorous diets are an inefficient means of obtaining energy; energy is limited, and most energy is lost between trophic levels. However, predatory birds (owls and eagles) and other smaller carnivores, including foxes and weasels, feed on the rodents. Larger carnivores, such as lynxes and wolves, prey on the larger animals. Omnivores, such as bears and raccoons are fairly common, sometimes picking through human garbage.
A considerable number of birds such as Siberian Thrush, White's Thrush and Dark-throated Thrush, migrate to this habitat to take advantage of the long summer days and abundance of insects found around the numerous bogs and lakes. Of the perhaps 300 species of birds that summer in the taiga, only 30 stay for the winter (Sayre, 28). These are either carrion-feeding or large raptors that can take live mammal prey, including Golden Eagle, Rough-legged Buzzard, and Raven, or else seed-eating birds, including several species of grouse and crossbills.
Fire
Fire is the dominant natural disturbance in the taiga, as well as being an important disturbance mechanism in many other forest types, such as temperate, sub-alpine and chaparral forests. Large, stand-replacing fires, particularly in the taiga, determine the age distribution and spatial age mosaic of the forested landscape.
Fire suppression
![]() | This section may contain material not related to the topic of the article and should be moved to On-topic instead. Please help improve this section or discuss this issue on the talk page. (Learn how and when to remove this message) |
![]() | It has been suggested that this article be merged into wildfire#Fire suppression. (Discuss) Proposed since September 2006. |
In North America, the belief that fire suppression has substantially reduced the average annual area burned is widely held by resource managers and is often thought to be self-evident. Direct empirical evidence however is essentially limited to just two studies by Stocks (1991) and Ward and Tithecott (1993), that use Ontario government fire records to make comparisons of average annual area burned between areas with and without aggressive fire suppression policies. Numerous subsequent studies have presented the same information, often in a different format (Martell 1994, Martell 1996, Weber & Stocks 1998, Li 2000, Ward & Mawdsley 2000). The proponents of these studies argue that areas without aggressive fire suppression policies have larger average fire sizes and greater average annual area burned and a longer interval between fires and that this is evidence of the effect of fire suppression.
However, the idea that fire suppression can effectively reduce the average annual area burned is the focus of a vocal debate in the scientific literature. In particular, several recent papers have argued against this idea (Miyanishi & Johnson 2001, Miyanishi et al. 2002, Bridge et al 2005). These papers claim that statistically rigorous techniques for estimating the average annual area burned, called the fire cycle, do not show changes in the fire cycle associated with fire suppression and that the evidence used to support the effect of fire suppression is biased and has been presented in a way that is flawed. Note that none of these papers criticize fire management agencies for being anything less than completely committed to their mandate. Nor do they suggest that fire personnel are not well trained, efficiently deployed or well managed. Instead, these papers simply suggest that despite the resources employed, fire management agencies are simply unable to effectively reduce the average annual area burned.
The impact that effective fire suppression may have on the average annual area burned is important for many reasons, but in particular, its impact is key to the current paradigm of sustainable forest management in many jurisdictions. One of the core aspects of SFM in many jurisdictions is the use of wood supply models to determine sustainable harvest levels. This determination of sustainable harvest levels often assumes that fire suppression has been effective at reducing the average annual area burned. Thus, if current assumptions about the effect of fire suppression are wrong, the impact on SFM could be substantial.
Evidence that fire suppression has been effective
For the most part, studies that support the effects of fire suppression compare either the number of fires or the average fire size between areas with and without aggressive fire suppression policies. Typically, these studies use the same or similar data from provincial fire records for Ontario covering a span of about 20 years.
Proponents of these studies have argued that, firstly, fires are, on average, much larger in areas without aggressive fire suppression policies than in areas with aggressive fire suppression policies because these fires are allowed to spread freely. Secondly, the proponents have argued that far more lightning caused fires are detected in areas with aggressive fire suppression and yet, the average annual area burned is much higher in areas without aggressive fire suppression. It is implied that fire suppression must, therefore, be reducing the area burned by lightning fires.
Recently, Cumming (2005) used novel approaches to analyze multiple components of fire management activity in greater detail than previously done, and confirmed the effectiveness of fire suppression.
Evidence that fire suppression has not been effective
On the other side of the debate, the evidence that fire suppression has not been effective at reducing the average annual area burned has come primarily in two forms. Firstly, in the form of time-since-fire studies, which, it has been argued, do not show detectable changes in the fire cycle that can be associated with fire suppression. Secondly, in the form of criticism of the way that provincial fire records have been used to support the effect of fire suppression.
Numerous time-since fire studies have been done in the temperate, boreal and sub-alpine forests across Canada and the U.S. (reviewed in Johnson 1992, see also Bergeron & Archambault 1993, Johnson & Wowchuck 1993, Johnson et al. 1995, Weir et al. 2000). The techniques used in these studies are felt to be well founded in statistical theory (Johnson & Van Wagner 1985, Johnson & Gutsell 1994, Reed 1994, Reed et al 1998) and recent improvements offer a way to statistically compare different periods of time to see if they possess significantly different fire cycles (Reed 1994, Reed et al 1998). While these studies often show a change in the fire cycle at the beginning of the 20th century, this change is usually associated with large-scale climatic factors, such as the end of the little ice age (Johnson 1992, Bergeron & Archambault 1993, Weir et al. 2000), and not fire suppression. In particular, around Ontario there have been at least six time-since-fire studies that show there has been no change in the fire cycle since 1920 (Heinselmann 1973, Woods & Day 1977, Suffling et al. 1982, Bergeron 1991, Gauthier et al. 2000, and Bridge et al. 2005). In Ontario, active fire suppression activities began sometime in the late 1910's, but these suppression activities are generally thought to be minimal compared with post 1950 when fire suppression began in earnest and technological advances made fire fighting much more effective (OMNR 2002, Thompson 2000).
Comparisons of the average annual area burned between areas with and without aggressive fire suppression policies, it is argued, are biased by the fact that small fires are virtually unreported in areas without aggressive fire suppression policies, where detection often relies on reports from settlements or commercial aircraft. Critics have argued that the number of lightning caused fires in areas with and without aggressive fire suppression policies are in fact quite similar and that the smaller average fire size, and the lower proportion of fires in larger size classes in areas with aggressive fire suppression is clearly a consequence of this bias (Miyanishi & Johnson 2001, Miyanishi et al. 2001).
Critics have also argued that despite suppression attempts the actual number of large fires in both areas is quite similar (Miyanishi & Johnson 2001, Miyanishi et al 2001). It has been argued that if fire suppression cannot impact the large fires, then it cannot impact the average annual area burned since almost all of the area is burned by only a few large fires.
Finally, studies that compare areas are also often based on averages of annual area burned made over periods of 12 to 17 years (Martell 1994, 1996, Ward & Tithecott 1993, Li 2000). Some have argued that this is too short a time period because the extreme year to year variation in area burned makes such averages highly variable and difficult to interpret (Johnson et al. 1996, Weir et al. 2000).
Reasons why fire suppression may not have affected the fire cycle
Several people (Weir et al. 1995, Johnson et al. 1995, 1998) have explored reasons why fire suppression may not have affected the fire cycle. In general, they feel that in closed-canopied forests, like the boreal, as little as 3% of the lightning caused fires account for up to 95% of the area burned (Stocks & Street 1993, Johnson & Wowchuck 1993). Most fires remain small, but a few occur under conditions that allow them to increase rapidly in size. It is this small proportion of large lightning caused fires which has the most influence on the area burned and the fire cycle.
In years with a large area burned, fires in these closed-canopied forests characteristically have high intensities, high rates of spread and high duff consumption. In these years, extreme fire behaviour is preceded by a persistent anomalous high pressure system which produces prolonged periods of above normal temperatures and below normal precipitation (Newark 1975, Harrington & Flannigan 1987), and leads to the severe drying of both medium and heavy fuels. Under these extreme conditions, fire behaviour exhibits little difference between aspect, elevation and vegetation type (Anderson 1968, Alexander et al. 1983, Nimchuck 1983, Janz & Nimchuck 1983, Street 1985, Flannigan & Harrington 1988, Fryer & Johnson 1988). In years with only a small area burned, differences in aspect, slope, elevation and vegetation composition can have a significant effect on the fire behaviour (Alexander & McAlpine 1987, Johnson et al 1998), however, the area burned in these years is insignificant.
The extreme fire behaviour associated with persistent high pressure systems results in large areas burned. It has been argued that during these years, it is unlikely that fire suppression can significantly influence the total area burned because under these conditions fire management agencies are quickly overwhelmed (Weir et al. 1995).
See also
References
- Arno, S. F. & Hammerly, R. P. 1984. Timberline. Mountain and Arctic Forest Frontiers. The Mountaineers, Seattle. ISBN 0-89886-085-7
- Arno, S. F., Worral, J., & Carlson, C. E. (1995). Larix lyallii: Colonist of tree-line and talus sites. Pp. 72-78 in Schmidt, W. C. & McDonald, K. J., eds., Ecology and Management of Larix Forests: A Look Ahead. USDA Forest Service General Technical Report GTR-INT-319.
- Sayre, A. P. (1994). Taiga. New York: Twenty-First Century Books. ISBN 0-8050-2830-7
BERGERON, Y. 1991. The influence of island and mainland lakeshore landscapes on boreal forest fire regimes. Ecology 72:1980-1992.
- BERGERON, Y., and S. ARCHAMBAULT. 1993. Decrease of forest fires in Quebec's southern boreal zone and its relation to global warming since the end of the Little Ice Age. Holocene 3:255-259.
- BRIDGE, S.R.J, K. MIYANISHI AND E.A. JOHNSON. 2005. A Critical Evaluation of Fire Suppression Effects in the Boreal Forest of Ontario. Forest Science 51:41-50.
- CUMMING, S.G. 2005. Effective fire suppression in boreal forests. Can. J. For. Res. 35: 772–786.
- HEINSELMAN, M.L. 1973. Fire in the virgin forests of the Boundary Waters Canoe Area, Minnesota. Quat. Res. 3:329-382.
- JOHNSON, E.A. 1992. Fire and vegetation dynamics: studies from the North American boreal forest. Cambridge University Press, Cambridge.
- JOHNSON, E.A., AND S.L. GUTSELL. 1994. Fire frequency models, methods and interpretations. Adv. Ecol. Res. 25:239-287.
- JOHNSON, E.A., AND D.R. WOWCHUK. 1993. Wildfires in the southern Canadian Rocky Mountains and their relationship to mid-troposhperic anomalies. Can. J. For. Res. 23:1213-1222.
- JOHNSON, E.A., AND C.E. VAN WAGNER. 1985. Theory and use of two fire history models. Can. J. For. Res. 15:214-220.
- JOHNSON, E.A., K. MIYANISHI, AND S.R.J. BRIDGE. 2001. Wildfire regime in the boreal forest and the idea of suppression and fuel buildup. Conserv. Biol. 15:1554-1557.
- JOHNSON, E.A., K. MIYANISHI, AND J.M.H. WEIR. 1995. Old-growth, disturbance, and ecosystem management. Can. J. Bot. 73:918-926.
- JOHNSON, E.A., K. MIYANISHI, and J.M.H. WEIR. 1996. Old-growth, disturbance, and ecosystem management: Reply. Can. J. Bot. 74:511.
- JOHNSON, E.A., K. MIYANISHI, AND J.M.H. Weir. 1998. Wildfires in the western Canadian boreal forest: Landscape patterns and ecosystem management. J. Veg. Sci. 9:603-610.
- LI, C. 2000. Fire regimes and their simulation with reference to Ontario. P. 115-140 in Ecology of a managed terrestrial landscape: patterns and processes of forest landscapes in Ontario, Perera, A.H., D.L. Euler, and I.D. Thompson (eds.). UBC Press, Vancouver, BC.
- MARTELL, D.L. 1994. The impact of fire on timber supply in Ontario. For. Chron. 70:164-173.
- MARTELL, D.L. 1996. Old-growth, disturbance, and ecosystem management: commentary. Can. J. Bot. 74:509-510.
- MIYANISHI, K., AND E.A. JOHNSON. 2001. A re-examination of the effects of fire suppression in the boreal forest. Can. J. For. Res. 31:1462-1466.
- MIYANISHI, K., S.R.J. BRIDGE, AND E.A. JOHNSON. 2002. Wildfire regime in the boreal forest. Conserv. Biol. 16:1177-1178.
- OMNR. 2002. Forest management guide for natural disturbance pattern emulation, Version 3.1. Ont. Min. Nat. Res., Queen’s Printer for Ontario, Toronto. ON. 40 p.
- REED, W.J. 1994. Estimating the historic probability of stand-replacement fire using the age-class distribution of undisturbed forest. For. Sci. 40:104-119.
- REED, W.J. 1998. Determining changes in historical forest fire frequency from a time-since-fire map. J. Agric. Biol. Env. Stat. 3:430-450.
- STOCKS, B.J. 1991. The extent and impact of forest fires in northern circumpolar countries. P. 197-202 in Global biomass burning: atmospheric, climatic and biospheric implications, Levine, J.S. (ed.). MIT Press, Cambridge, MA.
- STOCKS, B.J., AND R.B. STREET. 1983. Forest fire weather and wildfire occurrence in the boreal forest of northwestern Ontario. P. 249-265 in Resources and dynamics of the boreal zone, Wein, R.W., R.R. Riewe, and I.R. Methven (eds.). Association of Canadian Universities Northern Studies, Ottawa, ON.
- SUFFLING, R, B. SMITH, AND J. DAL MOLIN. 1982. Estimating past forest age distributions and disturbance rates in north-western Ontario: a demographic approach. J. Env. Manage. 14:45-56
- WARD, P.C., AND W. MAWDSLEY. 2000. Fire management in the boreal forests of Canada. P. 274-288 In Fire, climate change, and carbon cycling in the boreal forest, Kasischke, E.S., and B.J. Stocks (eds.). Springer, New York, NY.
- WARD, P.C., AND A.G. TITHECOTT. 1993. The impact of fire management on the boreal landscape of Ontario. Aviation, Flood and Fire Management Branch Publication No. 305. Ont. Min. Nat. Res., Queens Printer for Ontario, Toronto, ON.
- WEBER, M.G., AND B.J. STOCKS. 1998. Forest fires in the boreal forests of Canada. P. 215-233 in Large forest fires, Moreno, J.M. (ed.). Backhuys Publishers, Leiden, The Netherlands.
- WEIR, J.M.H., E.A. JOHNSON, AND K. MIYANISHI. 2000. Fire frequency and the spatial age mosaic of the mixed-wood boreal forest in western Canada. Ecol. Applic. 10:1162-1177.
- WOODS, G.T., AND R.T. DAY. 1977. A fire history study of Quetico Provincial Park. Rep. No. 4, Fire Ecology Study, Quetico Prov. Park. Ont. Min. Nat. Res., Toronto, ON.
External links
- Boreal Forests/Taiga (WWF)
- Terraformers Canadian Taiga Conservation Foundation
- Arctic and Taiga (Canadian Geographic)
- Biomes of the World: Coniferous Forest. Videocassette. Schlessinger media (2001).
- Coniferous Forest. Earth Observatory. NASA. .
- Dixon, D. Franklin Watts Picture Atlas Forests. London: Franklin Watts.
- Taiga Rescue Network (TRN) A network of NGOs, indigenous peoples or individuals that works to protect the boreal forests.
- Index of Boreal Forests/Taiga ecoregions at bioimages.vanderbilt.edu
- The Nature Conservancy and its partners work to protect the Canadian Boreal Forest
Template:Terrestrial biomes Template:Ecozones
Categories: