Revision as of 08:39, 6 February 2019 editChiswick Chap (talk | contribs)Autopatrolled, Extended confirmed users, Page movers, New page reviewers, Pending changes reviewers, Rollbackers296,915 edits →top: replace wl← Previous edit | Revision as of 11:52, 6 February 2019 edit undoDrlectin (talk | contribs)402 editsNo edit summaryTags: Mobile edit Mobile web editNext edit → | ||
Line 1: | Line 1: | ||
{{short description|Pore-forming toxin found in the ] ''Eisenia fetida''}} | {{short description|Pore-forming toxin found in the ] ''Eisenia fetida''}} | ||
'''Lysenin''' is a ] in the ]ic fluid of the ] '']''. | '''Lysenin''' is a ] (PFT) in the ]ic fluid of the ] '']''. | ||
==Monomer== | ==Monomer== |
Revision as of 11:52, 6 February 2019
Pore-forming toxin found in the earthworm Eisenia fetidaLysenin is a pore-forming toxin (PFT) in the coelomic fluid of the earthworm Eisenia fetida.
Monomer
Lysenin is a protein produced in the coelomocytes-leucocyte of animals with coelom-of Eisenia fetida. This protein was first isolated from the coelomic fluid by Yoshiyuki Sekizawa from Kobayashi group in 1996, who named the protein lysenin (lysis+Eisenia). Lysenin is relatively small with a molecular weight of 33 kDa (Figure 1). The X-ray structure of lysenin was first determined by de Colibus et al., and structurally classified as a member of the Aerolysin family, with which it shares structure and function. From a structural point of view, lysenin monomers are formed by a receptor binding domain (right globular part, Figure 1) and a Pore Forming Module (rest of the molecule, Figure 1); domains shared by the rest of Aerolysin family. Lysenin monomer shows three different sphingomyelin binding motifs in the receptor binding domain. The part of the monomer that forms part of the β-barrel have been clarify after obtaining Lysenin pore structure. At first, the so-called β-hairpin was alleged to be a small region in the vicinity of the Pore Forming Module (shown in green in Figure 1). Currently, it is known that the β-barrel is formed by a larger region (shown in yellow in Figure 1).
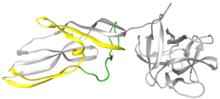
Membrane receptors
The natural membrane target of Lysenin is an animal plasma membrane lipid called Sphingomyelin, with at least three Phosphatidylcholines (PC) groups from sphingomyelin. Sphingomyelin is usually found associated to cholesterol in lipid rafts. Cholesterol, which enhances oligomerization, provides a stable platform with high lateral mobility where the monomer-monomer encounters are more probable. PFTs have shown to be able to remodel the membrane structure, sometimes even mixing lipid phases. In the case of lysenin, the detergent belt, the part of the β-barrel occupied by detergent in the Cryogenic Electron Microscopy (Cryo-EM) study of Lysenin pore, is 32 Å in height. On the other hand, sphingomyelin/Cholesterol bilayers are about 4.5 nm height. This difference in height between the detergent belt and the Sphingomyelin/Cholesterol bilayer implies a bend of the membrane in the region surrounding the pore, called negative mismatch. This bending results in a net attraction between pores that induce aggregation.
Binding, oligomerization and insertion
Membrane binding is a requisite to initiate PFT oligomerization. Lysenin monomers bind specifically to sphingomyelin via the receptor binding domain. The final Lysenin oligomer is constituted by nine monomers without quantified deviations, as shown for the first time by Munguira et al. Lysenin monomers bind to sphingomyelin-enriched domains, which provide a stable platform with a high lateral mobility, hence favouring the oligomerization. lysenin oligomerization occurs in a two-step process, as for proteins in general. The oligomerization process was recently imaged qualitatively.
First, the monomers adsorb to the membrane by specific interactions, resulting in monomers concentration increased. This increase is promoted by the small area where the membrane receptor accumulates owing to the fact that the majority of PFT membrane receptors are associated with lipid rafts. Another side effect, aside from the increase of monomer concentration, is the monomer-monomer interaction increase that boosts lysenin oligomerization. After a critical concentration is reached, several oligomers are formed simultaneously, as recently show by Munguira et al. Complete oligomers consist in nine monomers, although incomplete oligomerization process was also observed by Yilmaz et al. In contrast to PFT of cholesterol dependent cytolysin family, lysenin incomplete oligomer evolution to complete oligomers was never observed.
A complete oligomerization results in the so-called prepore state, placed on the membrane. Determining the structure by X-ray or Cryo-EM of the prepore is a challenging process that so far has not produce any results. The only available information about the prepore structure was provided by Atomic Force Microscopy (AFM). The measured prepore height was 90 Å; and the width 118 Å, with an inner pore of 50 Å (Figure 2). A model of the prepore, proposed by Podobnik et al., was built aligning monomer structure (PDB ID 3ZXD) with the pore structure (PDB ID 5GAQ) by their receptor-binding domains (residues 160 to 297). A recent study in aerolysin points out that the currently accepted model for the lysenin prepore should be revisited, according to the new available data on the aerolysin insertion. Another study has proved that lysenin prepore and prepore to pore models should be changed to fit recent observations.
A conformational change transforms the PFM to the transmembrane β-barrel, leading to the pore state. Such a conformational change produces a decrease in the oligomer height of 2.5 nm according to AFM measurements. The main dimensions, using lysenin pore X-ray structure, are height 97 Å, width 115 Å and the inner pore of 30 Å (Figure 2). Remarkably, the complete oligomerization is not a requisite for the insertion, although incomplete oligomers in the pore state can be found. The trigger mechanism for the prepore to pore transition in lysenin depends on three glutamic acids (E92, E94 and E97), and is activated by a decrease in pH, from physiological conditions to the acidic conditions reached after endocytosis. These three glutamic acids are located in an α-helix that forms part of the PFM, and are found in all aerolysin family members in its PFMs. The prepore to pore transition can be blocked if the membrane reaches certain density of oligomers, a mechanism first observed by Munguira et al., that could be general to all β-PFTs.

Insertion consequences
The ultimate consequences caused by lysenin pore formation are not well documented; however, there are threeplausible consequences:
- Punching the membrane breaks the sphingomyelin asymmetry between the two leaflets of the lipid bilayer, which cause apoptosis of the cell. PFTs are supposed to induce lipid flip-flop that can also break the sphingomyelin asymmetry.
- Increasing the calcium concentration in the cytoplasm, drives to apoptosis.
- Decreasing the potassium concentration in the cytoplasm causes apoptosis.
Biological role
The biological role of lysenin still remains unknown. It was suggested that lysenin can play a role as a defeat mechanism against attackers such as bacteria, fungi or small invertebrates. Nevertheless, sphingomyelin starts to appear in the plasma membrane of chordates. Therefore, lysenin, being dependent on sphingomyelin as a binding factor, cannot affect bacteria, fungi or in general invertebrates. Another hypothesis is that the earthworm, which is able to expel coelomic fluid under stress, generates an avoidance behaviour to its vertebrate predators (such as birds, hedgehogs or moles). If that is the case, the expelled lysenin might be more effective if the coelomic fluid reached the eye, where the concentration of sphingomyelin is ten times higher than in other body organs. A complementary hypothesis is that the coelomic fluid, exuded under stressful situations by the earthworm, which has a pungent smell -that gives name to the earthworm- was suggested to be an antipredator adaptation. It remains unknown whether (or not) lysenin plays a role generating an avoiding behaviour.
References
- Yilmaz, N., Yamaji-Hasegawa, A., Hullin-Matsuda, F. & Kobayashi, T. Molecular mechanisms of action of sphingomyelin-specific pore-forming toxin, lysenin. in Seminars in cell & developmental biology (Elsevier, 2017).
- SEKIZAWA, Y., HAGIWARA, K., NAKAJIMA, T. & KOBAYASHI, H. A novel protein, lysenin, that causes contraction of the isolated rat aorta: its purification from the coelomic fluid of the earthworm, Eisenia foetida. Biomedical Research 17, 197–203 (1996).
- De Colibus, L. et al. Structures of lysenin reveal a shared evolutionary origin for pore-forming proteins and its mode of sphingomyelin recognition. Structure 20, 1498–1507 (2012).
- De Colibus, L. et al. Structures of lysenin reveal a shared evolutionary origin for pore-forming proteins and its mode of sphingomyelin recognition. Structure 20, 1498–1507 (2012).
- Bokori-Brown, M. et al. Cryo-EM structure of lysenin pore elucidates membrane insertion by an aerolysin family protein. Nat Commun 7: 11293–11296. (2016).
- Ishitsuka, R. & Kobayashi, T. Cholesterol and lipid/protein ratio control the oligomerization of a sphingomyelin-specific toxin, lysenin. Biochemistry 46, 1495–1502 (2007).
- Simons, K. & Gerl, M. J. Revitalizing membrane rafts: new tools and insights. Nature reviews Molecular cell biology 11, nrm2977 (2010).
- Ishitsuka, R. & Kobayashi, T. Cholesterol and lipid/protein ratio control the oligomerization of a sphingomyelin-specific toxin, lysenin. Biochemistry 46, 1495–1502 (2007).
- Ros, U. & García-Sáez, A. J. More than a pore: the interplay of pore-forming proteins and lipid membranes. The Journal of membrane biology 248, 545–561 (2015).
- Yilmaz, N. & Kobayashi, T. Visualization of lipid membrane reorganization induced by a pore-forming toxin using high-speed atomic force microscopy. ACS nano 9, 7960–7967 (2015).
- Bokori-Brown, M. et al. Cryo-EM structure of lysenin pore elucidates membrane insertion by an aerolysin family protein. Nat Commun 7: 11293–11296. (2016).
- Quinn, P. J. Structure of sphingomyelin bilayers and complexes with cholesterol forming membrane rafts. Langmuir 29, 9447–9456 (2013).
- Guigas, G. & Weiss, M. Effects of protein crowding on membrane systems. Biochimica et Biophysica Acta (BBA)-Biomembranes 1858, 2441–2450 (2016).
- Munguira, I. L. B., Barbas, A. & Casuso, ignacio. Mechanism of blocking and unblocking of the pore formation of the toxin lysenin regulated by local crowdingThermometry at the nanoscale. Nanoscale 4, 4799–4829 (2018).
- De Colibus, L. et al. Structures of lysenin reveal a shared evolutionary origin for pore-forming proteins and its mode of sphingomyelin recognition. Structure 20, 1498–1507 (2012).
- Munguira, I. et al. Glasslike Membrane Protein Diffusion in a Crowded Membrane. ACS Nano 10, 2584–2590 (2016).
- Ishitsuka, R. & Kobayashi, T. Cholesterol and lipid/protein ratio control the oligomerization of a sphingomyelin-specific toxin, lysenin. Biochemistry 46, 1495–1502 (2007).
- Munguira, I. L. B., Barbas, A. & Casuso, ignacio. Mechanism of blocking and unblocking of the pore formation of the toxin lysenin regulated by local crowdingThermometry at the nanoscale. Nanoscale 4, 4799–4829 (2018).
- Lafont, F. & Van Der Goot, F. G. Bacterial invasion via lipid rafts. Cellular microbiology 7, 613–620 (2005).
- Munguira, I. L. B., Barbas, A. & Casuso, ignacio. Mechanism of blocking and unblocking of the pore formation of the toxin lysenin regulated by local crowdingThermometry at the nanoscale. Nanoscale 4, 4799–4829 (2018).
- Yilmaz, N. et al. Real-time visualization of assembling of a sphingomyelin-specific toxin on planar lipid membranes. Biophysical journal 105, 1397–1405 (2013).
- Mulvihill, E., van Pee, K., Mari, S. A., Müller, D. J. & Yildiz, Ö. Directly observing the lipid-dependent self-assembly and pore-forming mechanism of the cytolytic toxin listeriolysin O. Nano letters 15, 6965–6973 (2015).
- Yilmaz, N. et al. Real-time visualization of assembling of a sphingomyelin-specific toxin on planar lipid membranes. Biophysical journal 105, 1397–1405 (2013).
- Podobnik, M. et al. Crystal structure of an invertebrate cytolysin pore reveals unique properties and mechanism of assembly. Nature communications 7, 11598 (2016).
- Iacovache, M. I. et al. Cryo-EM structure of aerolysin variants reveals a novel protein fold and the pore-formation process. Nature communications 7, 12062 (2016).
- Munguira, I. L. B., Barbas, A. & Casuso, ignacio. Mechanism of blocking and unblocking of the pore formation of the toxin lysenin regulated by local crowdingThermometry at the nanoscale. Nanoscale 4, 4799–4829 (2018).
- Bokori-Brown, M. et al. Cryo-EM structure of lysenin pore elucidates membrane insertion by an aerolysin family protein. Nat Commun 7: 11293–11296. (2016).
- Yilmaz, N. et al. Real-time visualization of assembling of a sphingomyelin-specific toxin on planar lipid membranes. Biophysical journal 105, 1397–1405 (2013).
- Bokori-Brown, M. et al. Cryo-EM structure of lysenin pore elucidates membrane insertion by an aerolysin family protein. Nat Commun 7: 11293–11296. (2016).
- Yilmaz, N. et al. Real-time visualization of assembling of a sphingomyelin-specific toxin on planar lipid membranes. Biophysical journal 105, 1397–1405 (2013).
- Munguira, I. L. B., Takahashi, H., Casuso, I. & Scheuring, S. Lysenin Toxin Membrane Insertion Is pH-Dependent but Independent of Neighboring Lysenins. Biophysical Journal 113, 2029–2036 (2017).
- Munguira, I. L. B., Barbas, A. & Casuso, ignacio. Mechanism of blocking and unblocking of the pore formation of the toxin lysenin regulated by local crowdingThermometry at the nanoscale. Nanoscale 4, 4799–4829 (2018).
- Green, D. R. Apoptosis and sphingomyelin hydrolysis: the flip side. J Cell Biol 150, F5–F8 (2000).
- Ros, U. & García-Sáez, A. J. More than a pore: the interplay of pore-forming proteins and lipid membranes. The Journal of membrane biology 248, 545–561 (2015).
- Orrenius, S., Zhivotovsky, B. & Nicotera, P. Calcium: Regulation of cell death: the calcium–apoptosis link. Nature reviews Molecular cell biology 4, 552 (2003).
- Yu, S. P. Regulation and critical role of potassium homeostasis in apoptosis. Progress in neurobiology 70, 363–386 (2003).
- Ballarin, L. & Cammarata, M. Lessons in immunity: from single-cell organisms to mammals. (Academic Press, 2016).
- Kobayashi, H., Sekizawa, Y., Aizu, M. & Umeda, M. Lethal and non-lethal responses of spermatozoa from a wide variety of vertebrates and invertebrates to lysenin, a protein from the coelomic fluid of the earthworm Eisenia foetida. Journal of Experimental Zoology 286, 538–549 (2000).
- Sukumwang, N. & Umezawa, K. Earthworm-derived pore-forming toxin Lysenin and screening of its inhibitors. Toxins 5, 1392–1401 (2013).
- Kobayashi, H., Ohta, N. & Umeda, M. Biology of lysenin, a protein in the coelomic fluid of the earthworm Eisenia foetida. International Review of Cytology 236, 45–99 (2004).
- Swiderska, B. et al. Lysenin family proteins in earthworm coelomocytes–Comparative approach. Developmental & Comparative Immunology 67, 404–412 (2017).
- Berman, E. R. Biochemistry of the Eye. (Springer Science & Business Media, 2013).
- Edwards, C. A. & Bohlen, P. J. Biology and Ecology of Earthworms. (Springer Science & Business Media, 1996).