This is an old revision of this page, as edited by BSmith821 (talk | contribs) at 23:21, 13 July 2013. The present address (URL) is a permanent link to this revision, which may differ significantly from the current revision.
Revision as of 23:21, 13 July 2013 by BSmith821 (talk | contribs)(diff) ← Previous revision | Latest revision (diff) | Newer revision → (diff)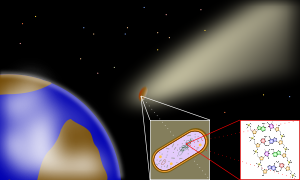
Panspermia (Template:Lang-el) is the hypothesis that life exists throughout the Universe, distributed by Comets,meteoroids, asteroids and planetoids.
It proposes that life forms that can survive the effects of space, such as extremophiles, become trapped in debris that is ejected into space after collisions between planets that harbor life and Small Solar System Bodies (SSSB). Microbes may travel dormant for an extended amount of time before colliding randomly with other planets or intermingling with protoplanetary disks. If met with ideal conditions on a new planet's surfaces, or inside a watery comet, the microbe becomes active and the process of evolution begins. Panspermia is not meant to address how life began, just the method that may cause its sustenance and propagation.
So Panspermia addresses the processes by which "Life is a Cosmic Phenomenon".
As a corollary, Panspermia proposes that life on Earth (and any life on other planets and exoplanets) was 'seeded' from space rather than arising through abiogenesis on that planet.
For clarification, the related but distinct idea of 'exogenesis' (Template:Lang-el) is a more limited hypothesis that proposes life on Earth was transferred from elsewhere in the Universe but makes no prediction about how widespread it is.
Hypothesis
The first known mention of the term was in the writings of the 5th century BC Greek philosopher Anaxagoras. In the nineteenth century it was again revived in modern form by several scientists, including Jöns Jacob Berzelius (1834), Kelvin (1871), Hermann von Helmholtz (1879) and, somewhat later, by Svante Arrhenius (1903). Charles Darwin is alleged to have been a proponent.
Sir Fred Hoyle (1915–2001) and Chandra Wickramasinghe (born 1939) were influential proponents of panspermia. In 1974 they proposed the hypothesis that some dust in interstellar space was largely organic (containing carbon), which Wickramasinghe later proved to be correct. Hoyle and Wickramasinghe further contended that life forms continue to enter the Earth's atmosphere, and may be responsible for epidemic outbreaks, new diseases, and the genetic novelty necessary for macroevolution. In a virtual presentation on April 7, 2009, physicist Stephen Hawking endorsed the hypothesis.
Panspermia does not necessarily suggest that life originated only once and subsequently spread through the entire Universe, but instead that once started, it may be able to spread to other environments suitable for replication. There is as yet no evidence to support or contradict panspermia, although the majority view holds that panspermia – especially in its interstellar form – is unlikely given the challenges of survival and transport in space.
Proposed mechanisms
The mechanisms proposed for interstellar panspermia are hypothetical and currently unproven. Panspermia can be said to be either interstellar (between star systems) or interplanetary (between planets in the same star system); its transport mechanisms may include radiation pressure and lithopanspermia (microorganisms in rocks). Interplanetary transfer of material is well documented, as evidenced by meteorites of Martian origin found on Earth. Space probes may also be a viable transport mechanism for interplanetary cross-pollination in our Solar System or even beyond. However, space agencies have implemented planetary protection procedures to reduce the risk of planetary contamination. Deliberate directed panspermia from space to seed Earth or sent from Earth to seed other solar systems have also been proposed. One twist to the hypothesis by engineer Thomas Dehel (2006), proposes that plasmoid magnetic fields ejected from the magnetosphere may move the few spores lifted from the Earth's atmosphere with sufficient speed to cross interstellar space to other systems before the spores can be destroyed. In 2012 mathematician Edward Belbruno and astronomers Amaya Moro-Martín and Renu Malhotra proposed that gravitational low energy transfer of rocks among the young planets of stars in their birth cluster is commonplace, and not rare in the general galactic stellar population.
Research
Until a large portion of the galaxy is surveyed for signs of life or contact is made with hypothetical extraterrestrial civilizations, the panspermia hypothesis in its fullest meaning will remain difficult to test.
Early life on Earth

The Precambrian fossil record indicates that life appeared soon after the Earth was formed. This would imply that life appeared within several hundred million years when conditions became favourable. Generally accepted scientific estimates of the age of the Earth place its formation (along with the rest of the Solar system) at about 4.55 billion years old. The oldest known sedimentary rocks are somewhat altered Hadean formations from the southern tip of Akilia Island, West Greenland. These rocks have been dated as no younger than 3.85 billion years.
The oldest known fossilized stromatolites or bacterial aggregates, are dated at 3.5 billion years old. The bacteria that form stromatolites, cyanobacteria, are photosynthetic. Most models of the origin of life have the earliest organisms obtaining energy from reduced chemicals, with the more complex mechanisms of photosynthesis evolving later. During the Late Heavy Bombardment of the Earth's Moon about 3.9 billion years (as evidenced by Apollo lunar samples) impact intensities may have been up to 100x those immediately before. From analysis of lunar melts and observations of similar cratering on Mars' highlands, Kring and Cohen suggest that the Late Heavy Bombardment was caused by asteroid impacts that affected the entire inner solar system. This is likely to have effectively sterilised Earth's entire planetary surface, including submarine hydrothermal systems that would be otherwise protected.
Extremophiles
Main article: ExtremophileAstrobiologists are particularly interested in studying extremophiles as many organisms of this type are capable of surviving in environments similar to those known to exist on other planets. Some organisms have been shown to be more resistant to extreme conditions than previously recognized, and may be able to survive for very long periods of time, probably even in deep space and, hypothetically, could travel in a dormant state between environments suitable for ongoing life.
Some bacteria and animals have been found to thrive in oceanic hydrothermal vents above 100 °C; a study revealed that a fraction of bacteria survive heating pulses up to 250°C in vacuo, while similar heating at normal atmospheric pressure leads to the total sterilization of samples. Other bacteria can thrive in strongly caustic environments, others at extreme pressures 11 km under the ocean, while others survive in extremely dry, desiccating conditions, frigid cold, vacuum or acid environments. Survival in space is not limited to bacteria, lichens or archea: the animal Tardigrade has been proven to survive the vacuum of space.
Experiments suggest that if bacteria were somehow sheltered from the radiation of space, perhaps inside a thick meteoroid or an icy comet, they could survive dormant for millions of years. Deinococcus radiodurans is a radioresistant bacterium that can survive high radiation levels. Duplicating the harsh conditions of cold interstellar space in their laboratory, NASA scientists have created primitive vesicles that mimic some aspects of the membraneous structures found in all living things. These chemical compounds may have played a part in the origin of life.
In 1995 scientists at California Polytechnic State University reported reviving bacteria that had been dormant for 25 million years.
On 26 April 2012, scientists reported that lichen survived and showed remarkable results on the adaptation capacity of photosynthetic activity within the simulation time of 34 days under Martian conditions in the Mars Simulation Laboratory (MSL) maintained by the German Aerospace Center (DLR).
On 17 March 2013, researchers reported data that suggested microbial life forms thrive in the Mariana Trench, the deepest spot on the Earth. Other researchers reported related studies that microbes thrive inside rocks up to 1900 feet below the sea floor under 8500 feet of ocean off the coast of the northwestern United States. According to one of the researchers,"You can find microbes everywhere — they're extremely adaptable to conditions, and survive wherever they are."
On 29 April 2013, French scientists reported that, during spaceflight inside the International Space Station, Pseudomonas aeruginosa bacteria seem to adapt to microgravity. The bacteria increased the number of viable cells, biofilm biomass, and thickness. Moreover, the biofilms formed during spaceflight exhibited a column-and-canopy structure that has not been observed on Earth.
Spores
Spores are another potential vector for transporting life through inhospitable and inimical environments, such as the depths of interstellar space. Spores are produced as part of the normal life cycle of many plants, algae, fungi and some protozoans, and some bacteria produce endospores or cysts during times of stress. These structures may be highly resilient to ultraviolet and gamma radiation, desiccation, lysozyme, temperature, starvation and chemical disinfectants, while metabolically inactive. Spores germinate when favourable conditions are restored after exposure to conditions fatal to the parent organism. Although computer models suggest that a captured meteoroid would typically take some tens of millions of years before collision with a neighboring solar system planet, there are documented viable Earthly bacterial spores that are 40 million years old that are very resistant to radiation, suggesting that lithopanspermia life-transfers are possible via meteorites exceeding 1m in size. Similarly, from the point of view of dynamical transport efficiency, life-bearing extrasolar asteroids could have been delivered to the Solar System via the weak transfer mechanism if life had a sufficiently early start in other planetary systems.
Potential habitats
Main articles: Astrobiology and Planetary habitabilityThe presence of past liquid water on Mars, suggested by river-like formations on the red planet, was confirmed by the Mars Exploration Rover missions. In December 2006, Michael C. Malin of Malin Space Science Systems published a paper in the journal Science which argued that his camera (the Mars Observer Camera) had found evidence suggesting water was occasionally flowing on the surface of Mars within the last five years.
Water oceans might exist on Europa, Enceladus, Triton and perhaps other moons in the Solar system. Even moons whose surface is mostly frozen ice may earlier have been melted internally by heat from radioactive rocky cores. Bodies like this may be common throughout the universe. Living bacteria found in ice core samples retrieved from 3,700 metres (12,100 ft) deep at Lake Vostok in Antarctica, have provided data for extrapolations to the likelihood of microorganisms surviving frozen in extraterrestrial habitats or during interplanetary transport. Also, bacteria have been discovered living within warm rock deep in the Earth's crust.
Extraterrestrial life
Main article: Extraterrestrial lifeEarth is the only place known by human beings to harbor life in the observed universe, while the sheer number of galaxies make it seem probable that life has arisen somewhere else in the Universe. According to current theories of physics, space travel over such vast distances would take an incredibly long time to the outside observer, with vast amounts of energy required. Nevertheless, small groups of researchers like the Search for Extra-Terrestrial Intelligence (SETI) continue to monitor the skies for transmissions from within our own galaxy at least.
Astrobiological proponents like the Rare Earth hypothesists claim that the conditions required for the evolution of intelligent life are probably exceedingly rare in the Universe, while simultaneously noting that simple single-celled microorganisms may well be abundant.
Spaceborne organic molecules and structures
A 2008 analysis of C/C isotopic ratios of organic compounds found in the Murchison meteorite indicates a non-terrestrial origin for these molecules rather than terrestrial contamination. Biologically relevant molecules identified so far include uracil, an RNA nucleobase, and xanthine. These results demonstrate that many organic compounds which are components of life on Earth were already present in the early Solar System and may have played a key role in life's origin.
In August 2009, NASA scientists identified one of the fundamental chemical building-blocks of life (the amino acid glycine) in a comet for the first time.
On August 8, 2011, a report, based on NASA studies with meteorites found on Earth, was published suggesting building blocks of DNA (adenine, guanine and related organic molecules) may have been formed extraterrestrially in outer space. In October 2011, scientists reported that cosmic dust contains complex organic matter ("amorphous organic solids with a mixed aromatic-aliphatic structure") that could be created naturally, and rapidly, by stars. One of the scientists suggested that these complex organic compounds may have been related to the development of life on Earth and said that, "If this is the case, life on Earth may have had an easier time getting started as these organics can serve as basic ingredients for life."
On August 29, 2012, and in a world first, astronomers at Copenhagen University reported the detection of a specific sugar molecule, glycolaldehyde, in a distant star system. The molecule was found around the protostellar binary IRAS 16293-2422, which is located 400 light years from Earth. Glycolaldehyde is needed to form ribonucleic acid, or RNA, which is similar in function to DNA. This finding suggests that complex organic molecules may form in stellar systems prior to the formation of planets, eventually arriving on young planets early in their formation.
In September 2012, NASA scientists reported that polycyclic aromatic hydrocarbons (PAHs), subjected to interstellar medium (ISM) conditions, are transformed, through hydrogenation, oxygenation and hydroxylation, to more complex organics - "a step along the path toward amino acids and nucleotides, the raw materials of proteins and DNA, respectively". Further, as a result of these transformations, the PAHs lose their spectroscopic signature which could be one of the reasons "for the lack of PAH detection in interstellar ice grains, particularly the outer regions of cold, dense clouds or the upper molecular layers of protoplanetary disks."
Still under investigation/undetermined
- Of the four Viking biological experiments performed by the Mars lander Viking in 1976, only the LR (Labeled Release) experiment gave results that were initially indicative of life (metabolism). However, the similar results from heated controls, how the release of indicative gas tapered off, and the lack of organic molecules in soil samples all suggest that the results were the result of a non-living chemical reaction rather than biological metabolism. Later experiments showed that existing oxidizers in the Martian soil could reproduce the results of the positive LR Viking experiment. Despite this, the Viking's LR experiment designer remains convinced that it is diagnostic for life on Mars.

- A meteorite originating from Mars known as ALH84001 was shown in 1996 to contain microscopic structures resembling small terrestrial nanobacteria. When the discovery was announced, many immediately conjectured that these were fossils and were the first evidence of extraterrestrial life — making headlines around the world. Public interest soon started to dwindle as most experts started to agree that these structures were not indicative of life, but could instead be formed abiotically from organic molecules. However, in November 2009, a team of scientists at Johnson Space Center, including David McKay, reasserted that there was "strong evidence that life may have existed on ancient Mars", after having reexamined the meteorite and finding magnetite crystals.
- On May 11, 2001, two researchers from the University of Naples claimed to have found live extraterrestrial bacteria inside a meteorite. Geologist Bruno D'Argenio and molecular biologist Giuseppe Geraci claim the bacteria were wedged inside the crystal structure of minerals, but were resurrected when a sample of the rock was placed in a culture medium. They believe that the bacteria were not terrestrial because they survived when the sample was sterilized at very high temperature and washed with alcohol. They also claim that the bacteria's DNA is unlike any on Earth. They presented a report on May 11, 2001, concluding that this is the first evidence of extraterrestrial life, documented in its genetic and morphological properties. Some of the bacteria they discovered were found inside meteorites that have been estimated to be over 4.5 billion years old, and were determined to be related to modern day Bacillus subtilis and Bacillus pumilis bacteria on Earth but appears to be a different strain.
- An Indian and British team of researchers led by Chandra Wickramasinghe reported on 2001 that air samples over Hyderabad, India, gathered from the stratosphere by the Indian Space Research Organization, contained clumps of living cells. Wickramasinghe calls this "unambiguous evidence for the presence of clumps of living cells in air samples from as high as 41 km, above which no air from lower down would normally be transported". Two bacterial and one fungal species were later independently isolated from these filters which were identified as Bacillus simplex, Staphylococcus pasteuri and Engyodontium album respectively. The experimental procedure suggested that these were not the result of laboratory contamination, although similar isolation experiments at separate laboratories were unsuccessful.
- A reaction report at NASA Ames indicated skepticism towards the premise that Earth life cannot travel to and reside at such altitudes. Max Bernstein, a space scientist associated with SETI and Ames, argues the results should be interpreted with caution, noting that "it would strain one's credulity less to believe that terrestrial organisms had somehow been transported upwards than to assume that extraterrestrial organisms are falling inward". Pushkar Ganesh Vaidya from the Indian Astrobiology Research Centre reported in his 2009 paper that "the three microorganisms captured during the balloon experiment do not exhibit any distinct adaptations expected to be seen in microorganisms occupying a cometary niche".
- In 2005 an improved experiment was conducted by ISRO. On April 10, 2005 air samples were collected from six places at different altitudes from the Earth ranging from 20 km to more than 40 km. Adequate precautions were taken to rule out any contamination from any microorganisms already present in the collection tubes. The samples were tested at two labs in India. The labs found 12 bacterial and 6 fungal colonies in these samples. The fungal colonies were Penicillium decumbens, Cladosporium cladosporioides, Alternaria sp. and Tilletiopsis albescens. Out of the 12 bacterial samples, three were identified as new species and named Janibacter hoyeli.sp.nov (after Fred Hoyle), Bacillus isronensis.sp.nov (named after ISRO) and Bacillus aryabhati (named after the ancient Indian mathematician, Aryabhata). These three new species showed that they were more resistant to UV radiation than similar bacteria found on Earth. For any organism living so far up the Earth's atmosphere or having come from outside Earth, the UV radiation resistance would be extremely critical for survival.

- Recent studies, applying the equivalent of Moore's Law to biological evolution and extrapolating backwards, propose that life began "9.7±2.5 billion years ago", billions of years before the Earth was formed. In the case of evolution, empirical evidence suggested a doubling of complexity every 376 million years. As the age of trees can be measured by the number of rings, the hypothesis that the age of life could be measured by biological complexity (i.e., the length of functional non-redundant DNA in the genome) was studied. If log-transformed complexity is plotted against the time of origin of large evolutionary lineages, then the points fit to a straight line (see figure). The exponential increase in complexity can be explained by a positive self-activating feed back loop. The regression line hits zero (i.e., one nucleotide) at "9.7±2.5 billion years ago". If this model is correct, and since our Solar System is 4.6 billion years ago, then life somehow arrived to Earth from older stellar systems. This hypothesis was criticized by Eugene Koonin who suggested that the rates of early biological evolution might have been much faster due to the absence of competition on early Earth. Chris Adami argued that "it is inconceivable that life began with just a few nucleotides" (see discussion). To answer this criticism, Sharov proposed a hypothetical abiogenesis scenario that starts from coenzyme-like molecules that are functionally equivalent to single nucleotides. (see Abiogenesis#Coenzyme world)
Disputed
A NASA research group found a small number of Streptococcus mitis bacteria living inside the camera of the Surveyor 3 spacecraft when it was brought back to Earth by Apollo 12. They believed that the bacteria survived since the time of the craft's launch to the Moon. However, these reports are disputed by Leonard D. Jaffe, who was Surveyor program scientist and custodian of the Surveyor 3 parts brought back from the Moon, stated in a letter to the Planetary Society that an unnamed member of his staff reported that a "breach of sterile procedure" took place at just the right time to produce a false positive result. NASA was funding an archival study in 2007 that was trying to locate the film of the camera-body microbial sampling to confirm the report of a breach in sterile technique. NASA currently stands by its original assessment: see Reports of Streptococcus mitis on the moon.
On January 10, 2013, Chandra Wickramasinghe reported in the fringe science Journal of Cosmology, of shapes resembling fossil diatom frustules in a new carbonaceous meteorite called Polonnaruwa that landed in the North Central Province of Sri Lanka on 29 December 2012. Early on, there was criticism that that Wickramasinghe's article was not an examination of the Polonnaruwa meteorite but of some terrestrial rock passed off as the meteorite.
Wickramasinghe's team remark that they are aware that a large number of unrelated stones have been submitted for analysis, and have no knowledge regarding the nature, source or origin of the stones their critics have examined, so Wickramasinghe clarifies that he is using the stones submitted by the Medical Research Institute in Sri Lanka.
In response to the criticism from other scientists, Wickramasinghe performed X-ray diffraction and isotope analyses to verify its meteoric origin. His analysis revealed a 95% silica and 3% quartz content, and interpreted this result as a "carbonaceous meteorite of unknown type". In addition, Wickramasinghe's team remarked that the temperature at which sand must be heated by lightning to melt and form a fulgurite (1770 °C) would have vaporized and burned all carbon-rich organisms and melted and thus destroyed the delicately marked silica frustules of the diatoms, and that the oxygen isotope data confirms its meteoric origin. Wickramasinghe's team also argues that since living diatoms require nitrogen fixation to synthetize amino acids, proteins, DNA, RNA and other life-critical biomolecules, a population of extraterrestrial cyanobacteria must have been a required component of the comet (Polonnaruwa meteorite) "ecosystem".
Hoaxes
A separate fragment of the Orgueil meteorite (kept in a sealed glass jar since its discovery) was found in 1965 to have a seed capsule embedded in it, whilst the original glassy layer on the outside remained undisturbed. Despite great initial excitement, the seed was found to be that of a European Juncaceae or Rush plant that had been glued into the fragment and camouflaged using coal dust. The outer "fusion layer" was in fact glue. Whilst the perpetrator of this hoax is unknown, it is thought he sought to influence the 19th century debate on spontaneous generation — rather than panspermia — by demonstrating the transformation of inorganic to biological matter.
Objections to panspermia and exogenesis
- Life as we know it requires the elements hydrogen, carbon, nitrogen, oxygen, iron, phosphorus and sulfur (H, C, N, O, Fe, P, and S respectively) to exist at sufficient densities and temperatures for the chemical reactions between them to occur. These conditions are not widespread in the Universe, so this limits the distribution of life as an ongoing process. First, the elements C, N and O are only created after at least one cycle of star birth/death: this is a limit to the earliest time life could have arisen. Second, densities of elements sufficient for the formation of more complex molecules necessary to life (such as amino acids) only occur in molecular dust clouds (10–10 particles/m), and (following their collapse) in solar systems. Third, temperatures must be lower than those in stars (elements are stripped of electrons: a plasma state) but higher than in the interstellar medium (reaction rates are too low). This restricts ongoing life to planetary environments where heavy elements are present at high densities, so long as temperatures are sufficient for plausible reaction rates. Note this does not restrict dormant forms of life to these environments, so this argument only contradicts the widest interpretation of panspermia — that life is ongoing and is spread across many different environments throughout the Universe — and presupposes that any life needs those elements, which the proponents of alternative biochemistries do not consider certain.
- Space is a damaging environment for life, as it would be exposed to radiation, cosmic rays and stellar winds. Environments may exist within meteors or comets that are somewhat shielded from these hazards. However, the extreme resistance of Deinococcus radiodurans to radiation, cold, dehydration and vacuum shows that at least one known organism is capable of surviving the hazards of space without need for special protection.
- Bacteria would not survive the immense heat and forces of an impact on Earth, also of the impact that launched them into space from their original habitat. However, most of the heat generated when a meteor enters the Earth's atmosphere is carried away by ablation and the interiors of freshly landed meteorites are rarely heated much and are often cold. The shock that sends them into space is the toughest challenge. Recent studies show that they could also survive if the impactor is larger or the planet has less gravity or less atmosphere. The existence of Martian meteorites and Lunar meteorites on Earth suggests material transfer from other celestial bodies to Earth happens regularly.
- Supporters of exogenesis also argue that on a larger scale, for life to emerge in one place in the Universe and subsequently spread to other planets would be simpler than similar life emerging separately on different planets. However, this assumes that the emergence of life in the entire Universe is rare enough as to limit it to one or few events or origination sites. Exogenesis still requires life to have originated from somewhere, most probably some form of abiogenesis. Given the immense expanse of the entire Universe, it has been argued that there is a higher probability that there exists (or has existed) another Earth-like planet that has yielded life than not. This explanation is more preferred under Occam's Razor than exogenesis since it theorizes that the creation of life is a matter of probability and can occur when the correct conditions are met rather than in exogenesis that assumes it is a singular event or that Earth did not meet those conditions on its own. In other words, exogenesis theorizes only one or few origins of life in the Universe, whereas abiogenesis theorizes that it is a matter of probability depending on the conditions of the celestial body. However, since to date no extraterrestrial life has been confirmed, both hypotheses still suffer from lack of information and many unidentified variables.
- Even if life were to survive - or develop in space — these would be very enduring life forms, as the hypothesis proposes, and they would have already visibly populated and altered Venus and Mars as well as other moons in the solar system.
Accidental panspermia
Thomas Gold a professor of astronomy suggested a "garbage theory" for the origin of life, the theory says that life on Earth might have spread from a pile of waste products accidentally dumped on Earth long ago by extraterrestrials.
Directed panspermia
Main article: Directed panspermiaDirected panspermia concerns the deliberate transport of microorganisms in space, sent to Earth to start life here, or sent from Earth to seed new solar systems with life. The Nobel prize winner Francis Crick, along with Leslie Orgel proposed seeds of life may have been purposely spread by an advanced extraterrestrial civilization, but considering an early "RNA world" Crick noted later that life may have originated on Earth.
Conversely, active directed panspermia has been proposed to secure and expand life in space. This may be motivated by biotic ethics that values, and seeks to propagate, the basic patterns of our organic gene/protein life-form. The panbiotic program would seed new solar systems nearby, and clusters of new stars in interstellar clouds. These young targets, where local life would not have formed yet, avoid any interference with local life.
For example, microbial payloads launched by solar sails at speeds up to 0.0001 c (30,000 m/s) would reach targets at 10 to 100 light-years in 0.1 million to 1 million years. Fleets of microbial capsules can be aimed at clusters of new stars in star-forming clouds, where they may land on planets or captured by asteroids and comets and later delivered to planets. Payloads may contain extremophiles for diverse environments and cyanobacteria similar to early microorganisms. Hardy multicellular organisms (rotifer cysts) may be included to induce higher evolution.
The probability of hitting the target zone can be calculated from
where A(target) is the cross-section of the target area, dy is the positional uncertainty at arrival; a - constant (depending on units), r(target) is the radius of the target area; v the velocity of the probe; (tp) the targeting precision (arcsec/yr); and d the distance to the target, guided by high-resolution astrometry of 1×10 arcsec/yr (all units in SIU). These calculations show that relatively near target stars(Alpha PsA, Beta Pictoris) can be seeded by milligrams of launched microbes; while seeding the Rho Ophiochus star-forming cloud requires hundreds of kilograms of dispersed capsules.Theoretically, unintended panspermia may occur by spacecraft travelling to other celestial bodies. This may concern space researchers who try to prevent contamination. However, directed panspermia may reach a few dozen target systems, leaving billions in the galaxy untouched for exploration. In any case, matter is exchanged meteor impacts in the solar system even without human intervention.
Directed panspermia to secure and expand life in space is becoming possible due to developments in solar sails, precise astrometry, extrasolar planets, extremophiles and microbial genetic engineering. After determining the composition of chosen meteorites, astroecologists performed laboratory experiments that suggest that many colonizing microorganisms and some plants could obtain many of their chemical nutrients from asteroid and cometary materials. However, the scientists noted that phosphate (PO4) and nitrate (NO3–N) critically limit nutrition to many terrestrial lifeforms. With such materials, and energy from long-lived stars, microscopic life planted by directed panspermia could find an immense future in the galaxy.
Missions
Foton-M3 spacecraft
In September 2007, after enduring a 12-day orbital mission and a fiery reentry, the European unmanned spacecraft Foton-M3 was retrieved from a field in Kazakhstan. The 5,500-pound capsule, seven feet in diameter, carried a payload of 43 European experiments in a range of scientific disciplines – including fluid physics, biology, crystal growth, radiation exposure and astrobiology. The capsule contained, among other things, lichen that were exposed to the radiation of space. Scientists also strapped basalt and granite disks riddled with cyanobacteria to the capsule's heat shield to see if the microorganisms could survive the brutal conditions of reentry. Some bacteria, lichens, spores, and even one animal (tardigrades) were found to have survived the outer space environment and cosmic radiation.
Living Interplanetary Flight Experiment
The Living Interplanetary Flight Experiment, which was developed by the Planetary Society, intended to send selected microorganisms on a three-year interplanetary round-trip in a small capsule aboard the Russian Fobos-Grunt spacecraft in 2011. The goal was to test whether organisms can survive a few years in deep space. Unfortunately, the spacecraft suffered technical difficulties soon after launch and fell back to Earth so the experiment was never carried out. The experiment would have tested one aspect of transpermia, the hypothesis that life could survive space travel, if protected inside rocks blasted by impact off one planet to land on another.
See also
3References
- Rampelotto, P. H. (2010). Panspermia: A promising field of research. In: Astrobiology Science Conference. Abs 5224.
- A variation of the panspermia hypothesis is necropanspermia which is described by astronomer Paul Wesson as follows: "The vast majority of organisms reach a new home in the Milky Way in a technically dead state ... Resurrection may, however, be possible." Grossman, Lisa (2010-11-10). "All Life on Earth Could Have Come From Alien Zombies". Wired. Retrieved 2010-11-10.
{{cite news}}
: Italic or bold markup not allowed in:|publisher=
(help) - Margaret O'Leary (2008) Anaxagoras and the Origin of Panspermia Theory, iUniverse publishing Group, # ISBN 978-0-595-49596-2
- Berzelius (1799-1848), J. J. "Analysis of the Alais meteorite and implications about life in other worlds".
{{cite journal}}
: Cite journal requires|journal=
(help)CS1 maint: numeric names: authors list (link) - Thomson (Lord Kelvin), W. (1871). "Inaugural Address to the British Association Edinburgh. "We must regard it as probably to the highest degree that there are countless seed-bearing meteoritic stones moving through space."". Nature. 4 (92): 261–278 . Bibcode:1871Natur...4..261.. doi:10.1038/004261a0.
- Arrhenius, S., Worlds in the Making: The Evolution of the Universe. New York, Harper & Row, 1908.
- MR. DARWIN ON DR. HAHN'S DISCOVERY OF FOSSIL ORGANISMS IN METEORITES.
- Wickramasinghe, D. T. & Allen, D. A. The 3.4-µm interstellar absorption feature. Nature 287, 518−519 (1980). Allen, D. A. & Wickramasinghe, D. T. Diffuse interstellar absorption bands between 2.9 and 4.0 µm. Nature 294, 239−240 (1981). Wickramasinghe, D. T. & Allen, D. A. Three components of 3–4 μm absorption bands. Astrophys. Space Sci. 97, 369−378 (1983).
- Fred Hoyle, Chandra Wickramasinghe and John Watson (1986). Viruses from Space and Related Matters. University College Cardiff Press.
- "Ruminations on other worlds". StatePress.com. April 7, 2009. Retrieved 2012-10-10.
{{cite news}}
:|first=
missing|last=
(help) - Weber, P; Greenberg, J. M. (1985). "Can spores survive in interstellar space?". Nature. 316 (6027): 403–407. Bibcode:1985Natur.316..403W. doi:10.1038/316403a0.
- Melosh, H. J. (1988). "The rocky road to panspermia". Nature. 332 (6166): 687–688. Bibcode:1988Natur.332..687M. doi:10.1038/332687a0. PMID 11536601.
- ^ C. Mileikowsky, F. A. Cucinotta, J. W. Wilson, B. Gladman, G. Horneck, L. Lindegren, J. Melosh, Hans Rickman, M. Valtonen, J. Q. Zheng (2000). "Risks threatening viable transfer of microbes between bodies in our solar system". Planetary and Space Science. 48 (11): 1107–1115. Bibcode:2000P&SS...48.1107M. doi:10.1016/S0032-0633(00)00085-4.
{{cite journal}}
: CS1 maint: multiple names: authors list (link) - Studies Focus On Spacecraft Sterilization
- European Space Agency: Dry heat sterilisation process to high temperatures
- ^ Crick, F. H.; Orgel, L. E. (1973). "Directed Panspermia". Icarus. 19: 341–348. doi:10.1016/0019-1035(73)90110-3+[http://adsabs.harvard.edu/abs/1979JBIS...32..419M].
{{cite journal}}
: External link in
(help)|doi=
- Mautner, Michael N. (2000). Seeding the Universe with Life: Securing Our Cosmological Future (PDF). Washington D. C.: Legacy Books (www.amazon.com). ISBN 0-476-00330-X.
- Mautner, M; Matloff, G. (1979). "Directed panspermia: A technical evaluation of seeding nearby solar systems" (PDF). J. British Interplanetary Soc. 32: 419.
- ^ Mautner, M. N. (1997). "Directed panspermia. 3. Strategies and motivation for seeding star-forming clouds" (PDF). J. British Interplanetary Soc. 50: 93–102.
- ^ BBC Staff (23 August 2011). "Impacts 'more likely' to have spread life from Earth". BBC. Retrieved 2011-08-24.
- "Electromagnetic space travel for bugs? - space - 21 July 2006 - New Scientist Space". Space.newscientist.com. Retrieved 2009-08-20.
- Dehel, T. (2006-07-23). "Uplift and Outflow of Bacterial Spores via Electric Field". 36th COSPAR Scientific Assembly. Held 16–23 July 2006. 36. Adsabs.harvard.edu: 1. arXiv:hep-ph/0612311. Bibcode:2006cosp...36....1D.
- ^ "Chaotic Exchange of Solid Material between Planetary" (PDF). Astrobiology. 12 (8). 2012. doi:10.1089/ast.2012.0825. Retrieved 2013-06-19.
{{cite journal}}
:|first=
missing|last=
(help); Unknown parameter|coauthors=
ignored (|author=
suggested) (help) - Slow-moving rocks better odds that life crashed to Earth from space News at Princeton, Sep 24, 2012
- Schidlowski, M. (1988). "A 3,800-Million-Year Isotopic Record Of Life From Carbon In Sedimentary-Rocks". Nature. 333 (6171): 313–318. Bibcode:1988Natur.333..313S. doi:10.1038/333313a0.
{{cite journal}}
: Unknown parameter|month=
ignored (help) - Gilmour I, Wright I, Wright J 'Origins of Earth and Life', The Open University, 1997, ISBN 0-7492-8182-0
- Nisbet E (2000). "The realms of Archaean life". Nature. 405 (6787): 625–6. doi:10.1038/35015187. PMID 10864305.
{{cite journal}}
: Unknown parameter|month=
ignored (help) - Lepland A, van Zuilen M, Arrhenius G, Whitehouse M and Fedo C, Questioning the evidence for Earth's earliest life — Akilia revisited, Geology; January 2005; v. 33; no. 1; p. 77-79; doi:10.1130/G20890.1
- ^ Cohen BA, Swindle TD, Kring DA (2000). "Support for the lunar cataclysm hypothesis from lunar meteorite impact melt ages". Science. 290 (5497): 1754–6. Bibcode:2000Sci...290.1754C. doi:10.1126/science.290.5497.1754. PMID 11099411.
{{cite journal}}
: Unknown parameter|month=
ignored (help)CS1 maint: multiple names: authors list (link) - Kring DA, Cohen BA (2002) Cataclysmic bombardment throughout the inner solar system 3.9-4.0 Ga. J GEOPHYS RES-PLANET 107 (E2): art. no. 5009
- Pavlov AK, Shelegedin VN, Kogan VT, Pavlov AA, Vdovina MA, Tret'iakov AV (2007). "". Biofizika (in Russian). 52 (6): 1136–40. PMID 18225667.
{{cite journal}}
: CS1 maint: multiple names: authors list (link) - "Science/Nature | Life flourishes at crushing depth". BBC News. 2005-02-04. Retrieved 2009-08-20.
- ^ Experiment lithopanspermia: test of interplanetary transfer and re-entry process of epi- and endolithic microbial communities in the FOTON-M3 Mission
- ^ "LIFE IN SPACE FOR LIFE ON EARTH - Biosatelite Foton M3". June 26, 2008. Retrieved 2009-10-13.
- ^ Jönsson, K. Ingemar Jönsson (9 September 2008). "Tardigrades survive exposure to space in low Earth orbit". Current Biology. 18 (17): R729 – R731. doi:10.1016/j.cub.2008.06.048. PMID 18786368. Retrieved 2009-10-13.
{{cite journal}}
: Unknown parameter|coauthors=
ignored (|author=
suggested) (help) - "Scientists Find Clues That Life Began in Deep Space :: Astrobiology Magazine - Earth science - evolution distribution Origin of life universe - life beyond". Astrobio.net. Retrieved 2009-08-20.
- Bacterium revived from 25 million year sleep Digital Center for Microbial Ecology
- Baldwin, Emily (26 April 2012). "Lichen survives harsh Mars environment". Skymania News. Retrieved 27 April 2012.
- de Vera, J.-P.; Kohler, Ulrich (26 April 2012). "The adaptation potential of extremophiles to Martian surface conditions and its implication for the habitability of Mars" (PDF). European Geosciences Union. Retrieved 27 April 2012.
- ^ Choi, Charles Q. (17 March 2013). "Microbes Thrive in Deepest Spot on Earth". LiveScience. Retrieved 17 March 2013.
- Glud, Ronnie; Wenzhöfer, Frank; Middleboe, Mathias; Oguri, Kazumasa; Turnewitsch, Robert; Canfield, Donald E.; Kitazato, Hiroshi (17 March 2013). "High rates of microbial carbon turnover in sediments in the deepest oceanic trench on Earth". Nature Geoscience. doi:10.1038/ngeo1773. Retrieved 17 March 2013.
- Oskin, Becky (14 March 2013). "Intraterrestrials: Life Thrives in Ocean Floor". LiveScience. Retrieved 17 March 2013.
- Tengra FK; et al. (29 April 2013). "Spaceflight Promotes Biofilm Formation by Pseudomonas aeruginosa". PLOS ONE. 8 (4): e6237. doi:10.1371/journal.pone.0062437. Retrieved 5 July 2013.
{{cite journal}}
: Explicit use of et al. in:|author=
(help)CS1 maint: unflagged free DOI (link) - The BIOPAN experiment MARSTOX II of the FOTON M-3 mission July 2008.
- Surviving the Final Frontier. 25 November 2002.
- "Detection, recovery, isolation, and characterization of bacteria in glacial ice and Lake Vostok accretion ice". Ohio State University. 2002. Retrieved 2011-02-04.
{{cite web}}
:|first=
missing|last=
(help)CS1 maint: multiple names: authors list (link) - Nanjundiah, V. (2000). "The smallest form of life yet?" (PDF). Journal of Biosciences. 25 (1): 9–10. doi:10.1007/BF02985175. PMID 10824192.
- See shrinking estimates of parameter values (since its inception in 1961) as discussed throughout the Drake equation article.
- Webb, Stephen, 2002. If the universe is teeming with aliens, where is everybody? Fifty solutions to the Fermi paradox and the problem of extraterrestrial life. Copernicus Books (Springer Verlag)
- Martins, Zita; Botta, Oliver; Fogel, Marilyn L.; Sephton, Mark A.; Glavin, Daniel P.; Watson, Jonathan S.; Dworkin, Jason P.; Schwartz, Alan W.; Ehrenfreund, Pascale (15 June 2008). "Extraterrestrial nucleobases in the Murchison meteorite". Earth and Planetary Science Letters. 270 (1–2): 130–136. arXiv:0806.2286. Bibcode:2008E&PSL.270..130M. doi:10.1016/j.epsl.2008.03.026.
- AFP Staff (20 August 2009). "We may all be space aliens: study". AFP. Retrieved 2011-08-14.
- Martins, Zita (Available online 20 March 2008). "Extraterrestrial nucleobases in the Murchison meteorite" (PDF). Earth and Planetary Science Letters. Retrieved 2008-10-07.
{{cite journal}}
: Check date values in:|date=
(help); Unknown parameter|coauthors=
ignored (|author=
suggested) (help) - "'Life chemical' detected in comet". NASA. BBC News. 18 August 2009. Retrieved 2010-03-06.
{{cite news}}
: Cite has empty unknown parameter:|coauthors=
(help) - Callahan, Michael P.; Smith, K.E.; Cleaves, H.J.; Ruzica, J.; Stern, J.C.; Glavin, D.P.; House, C.H.; Dworkin, J.P. (11 August 2011). "Carbonaceous meteorites contain a wide range of extraterrestrial nucleobases". PNAS. doi:10.1073/pnas.1106493108. Retrieved 2011-08-15.
- Steigerwald, John (8 August 2011). "NASA Researchers: DNA Building Blocks Can Be Made in Space". NASA. Retrieved 2011-08-10.
- ScienceDaily Staff (9 August 2011). "DNA Building Blocks Can Be Made in Space, NASA Evidence Suggests". ScienceDaily. Retrieved 2011-08-09.
- ^ Chow, Denise (26 October 2011). "Discovery: Cosmic Dust Contains Organic Matter from Stars". Space.com. Retrieved 2011-10-26.
- ScienceDaily Staff (26 October 2011). "Astronomers Discover Complex Organic Matter Exists Throughout the Universe". ScienceDaily. Retrieved 2011-10-27.
- Kwok, Sun; Zhang, Yong (26 October 2011). "Mixed aromatic–aliphatic organic nanoparticles as carriers of unidentified infrared emission features". Nature. 479 (7371): 80. Bibcode:2011Natur.479...80K. doi:10.1038/nature10542.
- Than, Ker (August 29, 2012). "Sugar Found In Space". National Geographic. Retrieved August 31, 2012.
- Staff (August 29, 2012). "Sweet! Astronomers spot sugar molecule near star". AP News. Retrieved August 31, 2012.
- Jørgensen, J. K. (2012). "Detection of the simplest sugar, glycolaldehyde, in a solar-type protostar with ALMA" (PDF). eprint.
{{cite journal}}
: Cite journal requires|journal=
(help); Unknown parameter|coauthors=
ignored (|author=
suggested) (help) - ^ Staff (September 20, 2012). "NASA Cooks Up Icy Organics to Mimic Life's Origins". Space.com. Retrieved September 22, 2012.
- ^ Gudipati, Murthy S.; Yang, Rui (September 1, 2012). "In-Situ Probing Of Radiation-Induced Processing Of Organics In Astrophysical Ice Analogs—Novel Laser Desorption Laser Ionization Time-Of-Flight Mass Spectroscopic Studies". The Astrophysical Journal Letters. 756 (1). doi:10.1088/2041-8205/756/1/L24. Retrieved September 22, 2012.
- The Carnegie Institution Geophysical Laboratory Seminar, "Analysis of evidence of Mars life" held 14 May 2007; Summary of the lecture given by Gilbert V. Levin, Ph.D. http://arxiv.org/abs/0705.3176, published by Electroneurobiología vol. 15 (2), pp. 39–47, 2007
- "New Study Adds to Finding of Ancient Life Signs in Mars Meteorite". NASA. 2009-11-30. Retrieved 2009-12-01.
- Thomas-Keprta, K., S. Clemett, D. McKay, E. Gibson and S. Wentworth (2009). "Origin of Magnetite Nanocrystals in Martian Meteorite ALH84001". Geochimica et Cosmochimica Acta (73): 6631–6677.
{{cite journal}}
: CS1 maint: multiple names: authors list (link) - "Alien visitors - 11 May 2001 - New Scientist Space". Space.newscientist.com. Retrieved 2009-08-20.
- D’Argenio, Bruno (March, 2001). "Microbes in rocks and meteorites: a new form of life unaffected by time, temperature, pressure". Rendiconti Lincei. 12 (1): 51–68. doi:10.1007/BF02904521. Retrieved 2009-10-13.
{{cite journal}}
: Check date values in:|date=
(help); Unknown parameter|coauthors=
ignored (|author=
suggested) (help) - http://www.lincei.it/pubblicazioni/rendicontiFMN/rol/pdf/S2001-01-04.pdf
- ^ "Scientists Say They Have Found Extraterrestrial Life in the Stratosphere But Peers Are Skeptical: Scientific American". Sciam.com. 2001-07-31. Retrieved 2009-08-20.
- Narlikar JV, Lloyd D, Wickramasinghe NC; et al. (2003). "Balloon experiment to detect micro-organisms in the outer space". Astrophys Space Science. 285 (2): 555–62. Bibcode:2003Ap&SS.285..555N. doi:10.1023/A:1025442021619.
{{cite journal}}
: Explicit use of et al. in:|author=
(help)CS1 maint: multiple names: authors list (link) - M. Wainwright, N.C. Wickramasinghe, J.V. Narlikar, P. Rajaratnam. "Microorganisms cultured from stratospheric air samples obtained at 41km". Retrieved 2007-05-11.
{{cite web}}
: CS1 maint: multiple names: authors list (link)Wainwright M (2003). "A microbiologist looks at panspermia". Astrophys Space Science. 285 (2): 563–70. Bibcode:2003Ap&SS.285..563W. doi:10.1023/A:1025494005689. - By Richard StengerCNN.com Writer (2000-11-24). "Space - Scientists discover possible microbe from space". Retrieved 2009-08-20.
{{cite news}}
:|author=
has generic name (help) - "Critique on Vindication of Panspermia" (PDF). Apeiron. 16 (3). July 2009. Retrieved 2009-11-28.
{{cite journal}}
:|first=
missing|last=
(help); Cite has empty unknown parameter:|coauthors=
(help) - Mumbai scientist challenges theory that bacteria came from space
- Janibacter hoylei sp. nov., Bacillus isronensis sp. nov. and Bacillus aryabhattai sp. nov., isolated from cryotubes used for collecting air from upper atmosphere. International Journal of Systematic and Evolutionary Microbiology 2009. http://ijs.sgmjournals.org/cgi/content/abstract/ijs.0.002527-0v1
- Discovery of New Microorganisms in the Stratosphere
- ^ Sharov, Alexei A.; Gordon, Richard (28 March 2013). "Life Before Earth" (PDF). arXiv. arXiv:1304.3381v1. Retrieved 16 April 2013.
- ^ Sharov, Alexei A. (12 June 2006). "Genome increase as a clock for the origin and evolution of life". Biology Direct. 1: 1–17. doi:10.1186/1745-6150-1-17. PMC 1526419.
{{cite journal}}
: CS1 maint: unflagged free DOI (link) - The date is based on the oldest inclusions found to date in meteorites, and is thought to be the date of the formation of the first solid material in the collapsing nebula.
Bouvier, A.; Wadhwa, M. (22 August 2010). "The age of the solar system redefined by the oldest Pb-Pb age of a meteoritic inclusion". Nature Geoscience. 3: 637–641. doi:10.1038/NGEO941. Retrieved 17 April 2013. - Koonin, E. V. (2002). Sequence - Evolution - Function: Computational Approaches in Comparative Genomics. Springer Verlag.
{{cite book}}
: Unknown parameter|coauthors=
ignored (|author=
suggested) (help) - Sharov, A.A. (22 April 2009). "Coenzyme autocatalytic network on the surface of oil microspheres as a model for the origin of life". International Journal of Molecular Sciences. 10: 1838–1852.
- Raffaelli, Nadia (2011). Origins of Life: The Primal Self-Organization - Nicotinamide Coenzyme Synthesis: A Case of Ribonucleotide Emergence or a Byproduct of the RNA World?. Springer Berlin Heidelberg. pp. 185–208. doi:10.1007/978-3-642-21625-1_9. ISBN 978-3-642-21624-4. Retrieved 17 April 2013.
- "Apollo 12 Mission". Lunar and Planetary Institute. Retrieved 2008-02-15.
- "Apollo 12 Remembered". Astrobiology Magazine (online 21 Nov 2004). Retrieved 2011-02-05.
- Wickramasinghe, N.C. (January 10, 2013). "Fossil Diatoms in a New Carbonaceous Meteorite" (PDF). 21 (37). Journal of Cosmology: 1–14. Retrieved January 14, 2013.
{{cite journal}}
: Cite journal requires|journal=
(help); Unknown parameter|coauthors=
ignored (|author=
suggested) (help) - "No, Diatoms Have Not Been Found in a Meteorite". Slate.com - Astronomy. 15 January 2013. Retrieved 2013-01-16.
{{cite news}}
:|first=
missing|last=
(help); Check|first=
value (help) - ^ "The Polonnaruwa meteorite: oxygen isotope, crystalline and biological composition" (PDF). Journal of Cosmology. 22 (2). 5 March 2013. Retrieved 2013-03-07.
{{cite journal}}
:|first=
missing|last=
(help); Unknown parameter|coauthors=
ignored (|author=
suggested) (help) - ^ N.C. Wickramasinghe, N.C. (4 February 2013). "Authenticity of the life-bearing Polonnaruwa meteorite" (PDF). Journal of Cosmology. Retrieved 2013-02-04.
{{cite journal}}
: Unknown parameter|coauthors=
ignored (|author=
suggested) (help) - Edward Anders, Eugene R. DuFresne,Ryoichi Hayatsu, Albert Cavaille, Ann DuFresne, and Frank W. Fitch. "Contaminated Meteorite," Science, New Series, Volume 146, Issue 3648 (Nov.27, 1964), 1157-1161.
- Life's Rocky Road Between Worlds, Space Daily, June 12, 2001
- "Science/Nature| Worms survived Columbia disaster". BBC News. 2003-05-01. Retrieved 2009-08-20.
- Survival of bacteria exposed to extreme acceleration: implications for panspermiaEarth and Planetary Science Letters 189 (2001) 1-8
- Gold, T. "Cosmic Garbage," Air Force and Space Digest, 65 (May 1960).
- "Anticipating an RNA world. Some past speculations on the origin of life: where are they today?" by L. E. Orgel and F. H. C. Crick in FASEB J. (1993) Volume 7 pages 238-239.
- Mautner, Michael N. (2009). "Life-centered ethics, and the human future in space" (PDF). Bioethics. 23: 433–440. doi:10.1111/j.1467-8519.2008.00688.x. PMID 19077128.
- Mautner, Michael Noah Ph.D. (2000). Seeding the Universe with Life: Securing our Cosmological Future (PDF). Legacy Books (www.amazon.com). ISBN 0-476-00330-X.
- ^ Mautner, Michael N. (2002). "Planetary bioresources and astroecology. 1. Planetary microcosm bioessays of Martian and meteorite materials: soluble electrolytes, nutrients, and algal and plant responses" (PDF). Icarus. 158: 72–86. Bibcode:2002Icar..158...72M. doi:10.1006/icar.2002.6841.
- Mautner, Michael N. (2005). "Life in the cosmological future: Resources, biomass and populations" (PDF). Journal of the British Interplanetary Society. 58: 167–180.
- "Foton-M3 experiments return to Earth". Retrieved 2007-09-26.
- NASA Collaborates With Russia On Foton-M3 Mission Sep 12, 2007.
- "LIFE Experiment". Planetary.org. Retrieved 2009-08-20.
- "Living interplanetary flight experiment: an experiment on survivability of microorganisms during interplanetary transfer" (PDF). Retrieved 2009-08-20.
- Crick F, 'Life, Its Origin and Nature', Simon and Schuster, 1981, ISBN 0-7088-2235-5
- Hoyle F, 'The Intelligent Universe', Michael Joseph Limited, London 1983, ISBN 0-7181-2298-4
Further reading
- Nature News. doi:10.1038/news040216-20.
{{cite journal}}
: Missing or empty|title=
(help) - Warmflash, D.; Weiss, B. (24 October 2005). "Did Life Come from Another World?". Scientific American.
External links
- Francis Crick's notes for a lecture on directed panspermia, dated 5 November 1976.
Origin of life | |
---|---|
History of research |
|
Prebiotic synthesis | |
Protocells | |
Earliest organisms | |
Research |
Extraterrestrial life | |||||||
---|---|---|---|---|---|---|---|
Events and objects |
| ||||||
Signals of interest |
| ||||||
Life in the Universe | |||||||
Planetary habitability |
| ||||||
Space missions |
| ||||||
Interstellar communication |
| ||||||
Types of alleged extraterrestrial beings | |||||||
Hypotheses | |||||||
Fermi paradox solutions | |||||||
Related topics |
|