This is an old revision of this page, as edited by Robertinventor (talk | contribs) at 13:35, 25 May 2013 (→Phoenix: added the 2010 results about oxygen isotope ratios suggesting reasonably abundant liquid water on the Mars surface recently). The present address (URL) is a permanent link to this revision, which may differ significantly from the current revision.
Revision as of 13:35, 25 May 2013 by Robertinventor (talk | contribs) (→Phoenix: added the 2010 results about oxygen isotope ratios suggesting reasonably abundant liquid water on the Mars surface recently)(diff) ← Previous revision | Latest revision (diff) | Newer revision → (diff) For the music group, see Water on Mars (band). For the Doctor Who episode, see The Waters of Mars.
![]() | This article may be too long to read and navigate comfortably. Consider splitting content into sub-articles, condensing it, or adding subheadings. Please discuss this issue on the article's talk page. (August 2011) |

Water on Mars exists almost exclusively as water ice. The Martian polar ice caps consist primarily of water ice, and further ice is contained in Martian surface rocks at more temperate latitudes (permafrost). A small amount of water vapor is present in the atmosphere. There are no bodies of liquid water on the Martian surface.
Current conditions on the planet surface do not support the long-term existence of liquid water. The average atmospheric pressure and temperature are far too low, leading to immediate freezing and resulting sublimation. Despite this, research suggests that in the past there was liquid water flowing on the surface, creating large areas similar to Earth's oceans. According to Steve Squyres, Principal investigator of the Mars Exploration Rover Missions (MER): "The idea been resolved. It's been resolved by Spirit, it's been resolved by Opportunity, it's been resolved by Curiosity, it's been amply resolved from orbit as well."
There are a number of direct and indirect proofs of water's presence either on or under the surface, e.g. stream beds, polar caps, spectroscopic measurement, eroded craters or minerals directly connected to the existence of liquid water (such as goethite), grey, crystalline hematite, phyllosilicates, opal, and sulfate. With the improved cameras on advanced Mars orbiters such as Viking, Mars Odyssey, Mars Global Surveyor, Mars Express, and the Mars Reconnaissance Orbiter pictures of ancient lakes, ancient river valleys, and widespread glaciation have accumulated. Besides the visual confirmation of water from a huge collection of images, an orbiting Gamma Ray Spectrometer found ice just under the surface of much of the planet. Also, radar studies discovered pure ice in formations that were thought to be glaciers. The Phoenix lander exposed ice as it landed, watched chunks of ice disappear, detected snow falling, and even saw drops of liquid water.
Today, it is generally believed that Mars had abundant water very early in its history during which snow and rain fell on the planet and created rivers, lakes, and possibly oceans. Large clay deposits were produced. Life may even have come into existence. Large areas of liquid water have disappeared, but climate changes have frequently deposited large amounts of water-rich materials in mid-latitudes. From these materials, glaciers and other forms of frozen ground came to be. Small amounts of water probably melt on steep slopes from time to time and produce gullies. Recent images have also detected yearly changes on some slopes that may have been caused by liquid water. Although Mars is very cold at present, water could exist as a liquid if it contains salts. Salt is expected to be on the Martian surface.
Details of how water has been discovered can be found in the sections that follow on the various orbiting and landing robots that have been sent to Mars. In addition, many bits and pieces of indirect evidence are listed here. Since several missions (Mars Odyssey, Mars Global Surveyor, Mars Reconnaissance Orbiter, Mars Express, Mars Opportunity Rover and Mars Curiosity Rover) are still sending back data from the Red Planet, discoveries continue to be made. One recent discovery, announced by NASA scientists on September 27, 2012, is that the Curiosity Rover found evidence for an ancient streambed suggesting a "vigorous flow" of water on Mars.
Image maps of Mars
The following imagemap of the planet Mars has embedded links to geographical features in addition to the noted Rover and Lander locations. Click on the features and you will be taken to the corresponding article pages. North is at the top; Elevations: red (higher), yellow (zero), blue (lower).



















Map of quadrangles
The following imagemap of the planet Mars is divided into the 30 quadrangles defined by the United States Geological Survey The quadrangles are numbered with the prefix "MC" for "Mars Chart." Click on the quadrangle and you will be taken to the corresponding article pages. North is at the top; 0°N 180°W / 0°N 180°W / 0; -180 is at the far left on the equator. The map images were taken by the Mars Global Surveyor.



Findings from probes
Main article: Timeline of discoveries of water on MarsMariner 9

Mariner 9 imaging revealed the first direct evidence of water in the form of river beds, canyons (including the Valles Marineris, a system of canyons over about 4,020 kilometres (2,500 mi) long), evidence of water erosion and deposition, weather fronts, fogs, and more. The findings from the Mariner 9 missions underpinned the later Viking program. The enormous Valles Marineris canyon system is named after Mariner 9 in honor of its achievements. Launched in 1971, its mission ended the following year.
Viking program
By discovering many geological forms that are typically formed from large amounts of water, Viking orbiters caused a revolution in our ideas about water on Mars. Huge river valleys were found in many areas. They showed that floods of water broke through dams, carved deep valleys, eroded grooves into bedrock, and traveled thousands of kilometers. Large areas in the southern hemisphere contained branched valley networks, suggesting that rain once fell. The flanks of some volcanoes are believed to have been exposed to rainfall because they resemble those occurring on Hawaiian volcanoes. Many craters look as if the impactor fell into mud. When they were formed, ice in the soil may have melted, turned the ground into mud, then the mud flowed across the surface. Normally, material from an impact goes up, then down. It does not flow across the surface, going around obstacles, as it does on some Martian craters. Regions, called "Chaotic Terrain,"seemed to have quickly lost great volumes of water which caused large channels to form downstream. The amount of water involved was almost unthinkable—estimates for some channel flows run to ten thousand times the flow of the Mississippi River. Underground volcanism may have melted frozen ice; the water then flowed away and the ground just collapsed to leave chaotic terrain.
The images below, some of the best from the Viking orbiters, are mosaics of many small, high resolution images.
-
Streamlined islands in Maja Valles suggest that large floods occurred on Mars.
-
Tear-drop shaped islands caused by flood waters from Maja Vallis. The islands are formed in the ejecta of Lod Crater, Bok Crater, and Gold Crater.
-
Large amounts of water would have been required to carry out the erosion shown in this image of Dromore Crater.
-
Networks of branched channels in Thaumasia quadrangle are strong evidence for rain on Mars in the past.
-
The ejecta from Arandas Crater acted like mud suggesting that large amounts of frozen water were melted by the impact.
-
Channels & troughs on the flank of Alba Patera. Some are associated with lava flows, others are probably caused by running water.

Results from Viking lander experiments strongly suggest the presence of water in the present and in the past of Mars. All samples heated in the gas chromatograph-mass spectrometer (GSMS) gave off water. However, the way the samples were handled prohibited an exact measurement of the amount of water. But, it was around 1%. General chemical analysis suggested the surface had been exposed to water in the past. Some chemicals in the soil contained sulfur and chlorine that were like those remaining after sea water evaporates. Sulfur was more concentrated in the crust on top of the soil, than in the bulk soil beneath. So it was concluded that the upper crust was cemented together with sulfates that were transported to the surface dissolved in water. This process is common on Earth's deserts. The sulfur may be present as sulfates of sodium, magnesium, calcium, or iron. A sulfide of iron is also possible.
Using results from the chemical measurements, mineral models suggest that the soil could be a mixture of about 80% iron-rich clay, about 10% magnesium sulfate (kieserite?), about 5% carbonate (calcite), and about 5% iron oxides (hematite, magnetite, goethite?). These minerals are typical weathering products of mafic igneous rocks. The presence of clay, magnesium sulfate, kieserite, calcite, hematite, and goethite strongly suggest that water was once in the area. Sulfate contains chemically bound water, hence its presence suggests water was around in the past. Viking 2 found similar group of minerals. Because Viking 2 was much farther north, pictures it took in the winter showed frost.
Mars Global Surveyor

The Mars Global Surveyor's Thermal Emission Spectrometer (TES) is an instrument able to detect mineral composition on Mars. Mineral composition gives information on the presence or absence of water in ancient times. TES identified a large (30,000 square-kilometer) area (in the Nili Fossae formation) that contained the mineral olivine. It is thought that the ancient impact that created the Isidis basin resulted in faults that exposed the olivine. Olivine is present in many mafic volcanic rocks; in the presence of water it weathers into minerals such as goethite, chlorite, smectite, maghemite, and hematite. The discovery of olivine is strong evidence that parts of Mars have been extremely dry for a long time. Olivine was also discovered in many other small outcrops within 60 degrees north and south of the equator. Olivine has been found in the SNC (shergottite, nakhlite, and chassigny) meteorites that are generally accepted to have come from Mars. Later studies have found that olivine-rich rocks cover more than 113,000 square kilometers of the Martian surface. That is 11 times larger than the five volcanoes on the Big Island of Hawaii.
On December 6, 2006 NASA released photos of two craters called Terra Sirenum and Centauri Montes which appear to show the presence of liquid water on Mars at some point between 1999 and 2001.
Hundreds of gullies have been discovered that were formed from liquid water, possible in recent times. These gullies occur on steep slopes and mostly in certain bands of latitude.
Below are some examples of gullies that were photographed by Mars Global Surveyor.
-
Group of gullies on north wall of crater that lies west of the crater Newton (41.3047 degrees south latitude, 192.89 east longitide). Image is located in the Phaethontis quadrangle.
-
Gullies in a crater in Eridania quadrangle, north of the large crater Kepler. Features that may be remains of old glaciers are present. One, to the right, has the shape of a tongue.
-
Gullies on one wall of Kaiser Crater. Gullies usually are found in only one wall of a crater. Location is Noachis quadrangle.
-
Full color image of gullies on wall of Gorgonum Chaos. Image is located in the Phaethontis quadrangle.
A few channels on Mars displayed inner channels that suggest sustained fluid flows. The most well-known is the one in Nanedi Valles. Another was found in Nirgal Vallis.
Many places on Mars show dark streaks on steep slopes, such as crater walls. Dark slope streaks have been studied since the Mariner and Viking missions. It seems that streaks start out being dark, then they become lighter with age. Often they originate with a small narrow spot, then widen and extend downhill for hundreds of meters. Streaks do not seem to be associated with any particular layer of material because they do not always start at a common level along a slope. Although many of the streaks appear very dark, they are only 10% or less darker than the surrounding surface. Mars Global Surveyor found that new streaks have formed in less than one year on Mars.
Several ideas have been advanced to explain the streaks. Some involve water, or even the growth of organisms. The generally accepted explanation for the streaks is that they are formed from the avalanching of a thin layer of bright dust that is covering a darker surface. Bright dust settles on all Martian surfaces after a period of time.
Dark streaks can be seen in the images below, as seen from Mars Global Surveyor.
-
Layers in Tikonravev Crater in Arabia. Layers may form from volcanoes, the wind, or by deposition under water. The craters on the left are pedestal craters. Dark slope streaks are seen to originate from certain layers (you may need to click on image to see the streaks).
-
Tikonravev Crater floor in Arabia quadrangle. Click on image to see dark slope streaks and layers.
-
Dark streaks in Diacria quadrangle.
-
Dark Streaks in Arabia quadrangle. Crater is about the size of Earth's Meteor Crater in Arizona.
Some parts of Mars show inverted relief. This occurs when materials are deposited on the floor of a stream then become resistant to erosion, perhaps by cementation. Later the area may be buried. Eventually erosion removes the covering layer. The former streams become visible since they are resistant to erosion. Mars Global Surveyor found several examples of this process. Many inverted streams have been discovered in various regions of Mars, especially in the Medusae Fossae Formation, Miyamoto Crater, and the Juventae Plateau.

Mars Pathfinder
Pathfinder found temperatures varied on a diurnal cycle. It was coldest just before sunrise (about −78 Celsius) and warmest just after Mars noon (about −8 Celsius). These extremes occurred near the ground which both warmed up and cooled down fastest. At this location, the highest temperature never reached the freezing point of water (0 °C), so Mars Pathfinder confirmed that where it landed it is too cold for liquid water to exist. However, water could exist as a liquid if it were mixed with various salts.
Surface pressures varied diurnally over a 0.2 millibar range, but showed 2 daily minima and two daily maxima. The average daily pressure decreased from about 6.75 millibars to a low of just under 6.7 millbars, corresponding to when the maximum amount of carbon dioxide had condensed on the south pole. The pressure on the Earth is generally close to 1000 millibars, so the pressure on Mars is very low. The pressures measured by Pathfinder would not permit water or ice to exist on the surface. But, if ice were insulated with a layer of soil, it could last a long time.
Other observations were consistent with water being present in the past. Some of the rocks at the Mars Pathfinder site leaned against each other in a manner geologists term imbricated. It is believed strong flood waters in the past pushed the rocks around until they faced away from the flow. Some pebbles were rounded, perhaps from being tumbled in a stream. Parts of the ground are crusty, maybe due to cementing by a fluid containing minerals.
There was evidence of clouds and maybe fog.
Mars Odyssey
Main article: Evidence of water on Mars from Mars OdysseyMars Odyssey found much evidence for water on Mars in the form of pictures and with a spectrometer it proved that much of the ground is loaded with ice. In July 2003, at a conference in California, it was announced that the Gamma Ray Spectrometer (GRS) on board the Mars Odyssey had discovered huge amounts of water over vast areas of Mars. Mars has enough ice just beneath the surface to fill Lake Michigan twice. In both hemispheres, from 55 degrees latitude to the poles, Mars has a high density of ice just under the surface; one kilogram of soil contains about 500 g of water ice. But, close to the equator, there is only 2 to 10% of water in the soil. Scientists believe that much of this water is locked up in the chemical structure of minerals, such as clay and sulfates. Previous studies with infrared spectroscopes have provided evidence of small amounts of chemically or physically bound water. The Viking landers detected low levels of chemically bound water in the Martian soil. It is believed that although the upper surface only contains a percent or so of water, ice may lie just a few feet deeper. Some areas, Arabia Terra, Amazonis quadrangle, and Elysium quadrangle contain large amounts of water. Analysis of the data suggest that the southern hemisphere may have a layered structure. Both of the poles showed buried ice, but the north pole had none close to it because it was covered over by seasonal carbon dioxide (dry ice). When the measurements were gathered, it was winter at the north pole so carbon dioxide had frozen on top of the water ice. There may be much more water further below the surface; the instruments aboard the Mars Odyssey are only able to study the top meter or so of soil. If all holes in the soil were filled by water, this would correspond to a global layer of water 0.5 to 1.5 km deep. The Phoenix lander confirmed the initial findings of the Mars Odyssey. It found ice a few inches below the surface and the ice is at least 8 inches deep. When the ice is exposed to the Martian atmosphere it slowly sublimates. In fact, some of the ice was exposed by the landing rockets of the craft.

Thousands of images returned from Odyssey support the idea that Mars once had great amounts of water flowing across its surface. Some pictures show patterns of branching valleys. Others show layers that may have formed under lakes. Deltas have been identified. For many years researchers believed that glaciers existed under a layer of insulating rocks. Lineated valley fill is one example of these rock-covered glaciers. They are found on the floors of some channels. Their surfaces have ridged and grooved materials that deflect around obstacles. Some glaciers on the Earth show such features. Lineated floor deposits may be related to Lobate debris aprons, which have been proven to contain large amounts of ice by orbiting radar. The pictures below, taken with the THEMIS instrument on board the Mars Odyssey, show examples of features that are associated with water present in the present or past.
- Reull Vallis with lineated floor deposits. Click on image to see relationship to other features. Floor deposits are believed to be formed from ice movement. Location is Hellas quadrangle. Reull Vallis with lineated floor deposits. Click on image to see relationship to other features. Floor deposits are believed to be formed from ice movement. Location is Hellas quadrangle.
-
Auqakuh Vallis. At one time a dark layer covered the whole area, now only a few pieces remain as buttes. Click on image to see layers. Layers may have formed from deposition on the bottom of lakes.
-
Channels near Warrego Valles. These branched channels are strong evidence for flowing water on Mars, perhaps during a much warmer period.
-
Semeykin Crater Drainage. Click on image to see details of beautiful drainage system. Location is Ismenius Lacus quadrangle.
-
Erosion features in Ares Vallis – the streamlined shape was probably formed by running water.
-
Delta in Lunae Palus quadrangle.
-
Athabasca Valles showing source of its water, Cerberus Fossae. Note streamlined islands which show direction of flow to south. Athabasca Valles is in the Elysium quadrangle.
-
Branching channels on floor of Melas Chasma. Image is in Coprates quadrangle.
-
Dao Vallis, as seen by THEMIS. Click on image to see relationship of Dao Vallis to other nearby features
Dao Vallis begins near a large volcano, called Hadriaca Patera, so it is thought to have received water when hot magma melted huge amounts of ice in the frozen ground. The partially circular depressions on the left side of the channel in the image above suggests that groundwater sapping also contributed water. In some areas large river valleys begin with a landscape feature called "Chaos" or Chaotic Terrain." It is thought that the ground collapsed, as huge amounts of water were suddenly released. Examples of Chaotic terrain, as imaged by THEMIS, are shown below.
-
Blocks in Aram showing possible source of water. The ground collapsed when large amounts of water were released. The large blocks probably still contain some water ice. Location is Oxia Palus quadrangle.
-
Huge canyons in Aureum Chaos. Click on image to see the gullies which may have formed from recent flows of water. Gullies are rare at this latitude. Location is Margaritifer Sinus quadrangle.
Phoenix
The Phoenix lander confirmed the existence of large amounts of water ice in the northern regions of Mars. This finding was predicted by theory. and was measured from orbit by the Mars Odyssey instruments. On June 19, 2008, NASA announced that dice-sized clumps of bright material in the "Dodo-Goldilocks" trench, dug by the robotic arm, had vaporized over the course of four days, strongly implying that the bright clumps were composed of water ice which sublimated following exposure. Even though dry ice also sublimates under the conditions present, it would do so at a rate much faster than observed.
On July 31, 2008, NASA announced that Phoenix confirmed the presence of water ice on Mars. During the initial heating cycle of a new sample, the Thermal and Evolved-Gas Analyzer's (TEGA) mass spectrometer detected water vapor when the sample temperature reached 0 °C. Liquid water cannot exist on the surface of Mars with its present low atmospheric pressure, except at the lowest elevations for short periods.
Results published in the journal Science after the mission ended reported that chloride, bicarbonate, magnesium, sodium potassium, calcium, and possibly sulfate were detected in the samples. Perchlorate (ClO4), a strong oxidizer, was confirmed to be in the soil. The chemical when mixed with water can greatly lower freezing points, in a manner similar to how salt is applied to roads to melt ice. Perchlorate may be allowing small amounts of liquid water to form on Mars today. Gullies, which are common in certain areas of Mars, may have formed from perchlorate melting ice and causing water to erode soil on steep slopes.
Additionally, during 2008 and early 2009, a debate emerged within NASA over the presence of 'blobs' which appeared on photos of the vehicle's landing struts, which have been variously described as being either water droplets or 'clumps of frost'. Due to the lack of consensus within the Phoenix science project, the issue had not been raised in any NASA news conferences. One scientist posited that the lander's thrusters splashed a pocket of brine from just below the Martian surface onto the landing strut during the vehicle's landing. The salts would then have absorbed water vapor from the air, which would have explained how they appeared to grow in size during the first 44 Martian days before slowly evaporating as Mars temperature dropped. Some images even suggest that some of the droplets darkened, then moved and merged; this is strong physical evidence that they were liquid.
-
Dice-sized clumps of bright material in the enlarged "Dodo-Goldilocks" trench vanished over the course of four days, implying that they were composed of ice which sublimated following exposure.
-
Color versions of the photos showing ice sublimation, with the lower left corner of the trench enlarged in the insets in the upper right of the images.
For about as far as the camera can see, the land is flat, but shaped into polygons between 2–3 meters in diameter and are bounded by troughs that are 20 cm to 50 cm deep. These shapes are due to ice in the soil expanding and contracting due to major temperature changes.
-
Comparison between polygons photographed by Phoenix on Mars...
-
... and as photographed (in false color) from Mars orbit...
-
... with patterned ground on Devon Island in the Canadian Arctic, on Earth.
The microscope showed that the soil on top of the polygons is composed of flat particles (probably a type of clay) and rounded particles. Clay is a mineral that forms from other minerals when water is available. So, finding clay proves the existence of past water. Ice is present a few inches below the surface in the middle of the polygons, and along its edges, the ice is at least 8 inches deep. When the ice is exposed to the Martian atmosphere it slowly sublimates.
Snow was observed to fall from cirrus clouds. The clouds formed at a level in the atmosphere that was around −65 °C, so the clouds would have to be composed of water-ice, rather than carbon dioxide-ice (dry ice) because the temperature for forming carbon dioxide ice is much lower—less than −120 °C. As a result of mission observations, it is now believed that water ice (snow) would have accumulated later in the year at this location. The highest temperature measured during the mission was −19.6 °C, while the coldest was −97.7 °C. So, in this region the temperature remained far below the freezing point (0°) of water. Bear in mind that the mission took place in the heat of the Martian summer.
Interpretation of the data transmitted from the craft was published in the journal Science. As per the peer reviewed data the site had a wetter and warmer climate in the recent past. Finding calcium carbonate in the Martian soil leads scientists to believe that the site had been wet or damp in the geological past. During seasonal or longer period diurnal cycles water may have been present as thin films. The tilt or obliquity of Mars changes far more than the Earth; hence times of higher humidity are probable. The data also confirms the presence of the chemical perchlorate. Perchlorate makes up a few tenths of a percent of the soil samples. Perchlorate is used as food by some bacteria on Earth. Another paper claims that the previously detected snow could lead to a buildup of water ice.
In 2010 an analysis of the isotope ratios of Oxygen in the Phoenix data suggested that "liquid water has been present on the Martian surface recently enough and abundantly enough to affect the composition of the current atmosphere".
Mars Rovers
The Mars Rovers Spirit and Opportunity found a great deal of evidence for past water on Mars. Designed to last only three months, both were still operating after more than six years. Although Spirit got trapped in a sand pit, Opportunity continues to provide scientific discovery.
The Spirit rover landed in what was thought to be a huge lake bed. However, the lake bed had been covered over with lava flows, so evidence of past water was initially hard to detect. As the mission progressed and the Rover continued to move along the surface more and more clues to past water were found.
On March 5, 2004, NASA announced that Spirit had found hints of water history on Mars in a rock dubbed "Humphrey". Raymond Arvidson, the McDonnell University Professor and chair of Earth and planetary sciences at Washington University in St. Louis, reported during a NASA press conference: "If we found this rock on Earth, we would say it is a volcanic rock that had a little fluid moving through it." In contrast to the rocks found by the twin rover Opportunity, this one was formed from magma and then acquired bright material in small crevices, which look like crystallized minerals. If this interpretation holds true, the minerals were most likely dissolved in water, which was either carried inside the rock or interacted with it at a later stage, after it formed.
By Sol 390 (Mid-February 2005), as Spirit was advancing towards "Larry's Lookout", by driving up the hill in reverse, it investigated some targets along the way, including the soil target, "Paso Robles", which contained the highest amount of salt found on the red planet. The soil also contained a high amount of phosphorus in its composition, however not nearly as high as another rock sampled by Spirit, "Wishstone". Squyres said of the discovery, "We're still trying to work out what this means, but clearly, with this much salt around, water had a hand here".
As Spirit traveled with a dead wheel in December 2007, pulling the dead wheel behind, the wheel scraped off the upper layer of the martian soil, uncovering a patch of ground that scientists say shows evidence of a past environment that would have been perfect for microbial life. It is similar to areas on Earth where water or steam from hot springs came into contact with volcanic rocks. On Earth, these are locations that tend to teem with bacteria, said rover chief scientist Steve Squyres. "We're really excited about this," he told a meeting of the American Geophysical Union (AGU). The area is extremely rich in silica – the main ingredient of window glass. The researchers have now concluded that the bright material must have been produced in one of two ways. One: hot-spring deposits produced when water dissolved silica at one location and then carried it to another (i.e. a geyser). Two: acidic steam rising through cracks in rocks stripped them of their mineral components, leaving silica behind. "The important thing is that whether it is one hypothesis or the other, the implications for the former habitability of Mars are pretty much the same," Squyres explained to BBC News. Hot water provides an environment in which microbes can thrive and the precipitation of that silica entombs and preserves them. Squyres added, "You can go to hot springs and you can go to fumaroles and at either place on Earth it is teeming with life – microbial life.
Opportunity rover was directed to a site that had displayed large amounts of hematite from orbit. Hematite often forms from water. When Opportunity landed, layered rocks and marble-like hematite concretions ("blueberries") were easily visible. In its years of continuous operation, Opportunity sent back much evidence that a wide area on Mars was soaked in liquid water.
During a press conference in March 2006, mission scientists discussed their conclusions about the bedrock, and the evidence for the presence of liquid water during their formation. They presented the following reasoning to explain the small, elongated voids in the rock visible on the surface and after grinding into it (see last two images below). These voids are consistent with features known to geologists as "vugs". These are formed when crystals form inside a rock matrix and are later removed through erosive processes, leaving behind voids. Some of the features in this picture are "disk-like", which is consistent with certain types of crystals, notably sulfate minerals. Additionally, mission members presented first data from the Mössbauer spectrometer taken at the bedrock site. The iron spectrum obtained from the rock El Capitan shows strong evidence for the mineral jarosite. This mineral contains hydroxide ions, which indicates the presence of water when the minerals were formed. Mini-TES data from the same rock showed that it consists of a considerable amount of sulfates. Sulfates also contain water.
Spirit Rover found evidence for water in the Columbia Hills of Gusev crater. In the Clovis group of rocks the Mossbauer spectrometer(MB) detected goethite. Goethite forms only in the presence of water, so its discovery is the first direct evidence of past water in the Columbia Hills's rocks. In addition, the MB spectra of rocks and outcrops displayed a strong decline in olivine presence, although the rocks probably once contained much olivine. Olivine is a marker for the lack of water because it easily decomposes in the presence of water. Sulfate was found, and it needs water to form. Other rock groups also contained sulfates. One type of soil, called Paso Robles, from the Columbia Hills, may be an evaporate deposit because it contains large amounts of sulfur, phosphorus, calcium, and iron. In addition, the MB found that much of the iron in Paso Robles soil was of the oxidized, Fe form, which would happen if water had been present.
After Spirit stopped working scientists studied old data from the Miniature Thermal Emission Spectrometer, or Mini-TES and confirmed the presence of large amounts of carbonate-rich rocks, which means that regions of the planet may have once harbored water. The carbonates were discovered in an outcrop of rocks called "Comanche."
On September 27, 2012, NASA scientists announced that the Curiosity rover found evidence for an ancient streambed suggesting a "vigorous flow" of water on Mars.
Evidence of water on Mars


On December 3, 2012, NASA reported that Curiosity performed its first extensive soil analysis, revealing the presence of water molecules, sulfur and chlorine in the Martian soil.
On March 18, 2013, NASA reported evidence from instruments on the Curiosity rover of mineral hydration, likely hydrated calcium sulfate, in several rock samples including the broken fragments of "Tintina" rock and "Sutton Inlier" rock as well as in veins and nodules in other rocks like "Knorr" rock and "Wernicke" rock. Analysis using the rover's DAN instrument provided evidence of subsurface water, amounting to as much as 4% water content, down to a depth of 60 cm (2.0 ft), in the rover's traverse from the Bradbury Landing site to the Yellowknife Bay area in the Glenelg terrain.
Mars Reconnaissance Orbiter
Main article: Evidence of water on Mars found by Mars Reconnaissance OrbiterThe Mars Reconnaissance Orbiter's HiRISE instrument has taken many images that strongly suggest that Mars has had a rich history of water-related processes. A major discovery was finding evidence of hot springs. These may have contained life and may now contain well-preserved fossils of life. Research, in the January 2010 issue of Icarus, described strong evidence for sustained precipitation in the area around Valles Marineris. The types of minerals there are associated with water. Also, the high density of small branching channels indicates a great deal of precipitation because they are similar to stream channels on the Earth.

-
Channels near the rim of Ius Chasma, as seen by HiRISE. The pattern and high density of these channels support precipitation as the source of the water. Location is Coprates quadrangle.
-
Example of inverted terrain in Parana Valles region, as seen by HiRISE under the HiWish program.
-
Inverted Stream Channels in Antoniadi Crater. Location is Syrtis Major quadrangle.
Some places on Mars show inverted relief. In these locations, a stream bed appears as a raised feature, instead of a depression. The inverted former stream channels may be caused by the deposition of large rocks or due to cementation of loose materials. In either case erosion would erode the surrounding land and consequently leave the old channel as a raised ridge because the ridge will be more resistant to erosion. Images below, taken with HiRISE show sinuous ridges that are old channels that have become inverted.
Using data from Mars Global Surveyor, Mars Odyssey and the Mars Reconnaissance Orbiter, scientists have found widespread deposits of chloride minerals. Usually chlorides are the last minerals to come out of solution. A picture below shows some deposits within the Phaethontis quadrangle. Evidence suggests that the deposits were formed from the evaporation of mineral-enriched waters. Lakes may have been scattered over large areas of the Martian surface. Carbonates, sulfates, and silica should precipitate out ahead of them. Sulfates and silica have been discovered by the Mars Rovers. Places with chloride minerals may have once held various life forms. Furthermore, such areas should preserve traces of ancient life.
Rocks on Mars have been found to frequently occur as layers, called strata, in many different places. Layers form by various ways. Volcanoes, wind, or water can produce layers. Many places on Mars show rocks arranged in layers. Scientists are happy about finding layers on Mars since layers may have formed under large bodies of water. Sometimes the layers display different colors. Light-toned rocks on Mars have been associated with hydrated minerals like sulfates. Instruments on orbiting spacecraft have detected clay (also called phyllosilicates) in some layers. Scientists are excited about finding hydrated minerals such as sulfates and clays on Mars because they are usually formed in the presence of water. Below are a few of the many examples of layers that have been studied with HiRISE.
-
Becquerel Crater layers. Click on image to see fault. Location is Oxia Palus quadrangle.
-
Close-up of layers in west slope of Asimov Crater. Shadows show the overhang. Some of the layers are much more resistant to erosion, so they stick out. Location is Noachis quadrangle.
-
Dark slope streaks near the top of a pedestal crater, as seen by HiRISE under the HiWish program.
Much of the surface of Mars is covered by a thick smooth mantle that is thought to be a mixture of ice and dust. This ice-rich mantle, a few yards thick, smoothes the land. But in places it displays a bumpy texture, resembling the surface of a basketball. Because there are few craters on this mantle, the mantle is relatively young. The images below, all taken with HiRISE, show a variety of views of this smooth mantle.
-
Niger Vallis with features typical of this latitude. Chevron pattern results from movement of ice-rich material. Click on image to see chevron pattern and mantle. Location is Hellas quadrangle.
-
Dissected Mantle with layers. Location is Noachis quadrangle.
-
Layers in mantle deposit, as seen by HiRISE, under the HiWish program. Mantle was probably formed from snow and dust falling during a different climate. Location is Thaumasia quadrangle.
The mantle is thought to result from frequent, major climate changes. Changes in Mars's orbit and tilt cause significant changes in the distribution of water ice from polar regions down to latitudes equivalent to Texas. During certain climate periods water vapor leaves polar ice and enters the atmosphere. The water returns to the ground at lower latitudes as deposits of frost or snow mixed generously with dust. The atmosphere of Mars contains a great deal of fine dust particles. Water vapor condenses on the particles, then they fall down to the ground due to the additional weight of the water coating. When ice at the top of the mantling layer goes back into the atmosphere, it leaves behind dust, which insulates the remaining ice. HiRISE has carried out many observations of gullies that are assumed to have been caused by recent flows of liquid water. Many gullies are imaged over and over to see if any changes occur. Some repeat observations of gullies have displayed changes that some scientists argue were caused by liquid water over the period of just a few years. Others say the flows were merely dry flows. These were first discovered by the Mars Global Surveyor. Below are some of the many hundreds of gullies that have been studied with HiRISE.
-
Gullies near Newton Crater, as seen by HiRISE, under the HiWish program. Place where there was an old glacier is labeled. Image from Phaethontis quadrangle.
-
Gullies near Newton Crater, as seen by HiRISE under the HiWish Program.
-
Gullies in a crater in Terra Sirenum, as seen by HiRISE under the HiWish Program.
-
Close-up of gully showing multiple channels and patterned ground, as seen by HiRISE under the HiWish program.
Of interest from the days of the Viking Orbiters are piles of material surrounding cliffs. These deposits of rock debris are called lobate debris aprons (LDAs). These features have a convex topography and a gentle slope from cliffs or escarpments; this suggests flow away from the steep source cliff. In addition, lobate debris aprons can show surface lineations just as rock glaciers on the Earth. In 2008, research with the Shallow Radar on the Mars Reconnaissance Orbiter provided strong evidence that the LDAs in Hellas Planitia and in mid northern latitudes are glaciers that are covered with a thin layer of rocks. Radar from the Mars Reconnaissance Orbiter gave a strong reflection from the top and base of LDAs, meaning that pure water ice made up the bulk of the formation (between the two reflections).). The discovery of water ice in LDAs demonstrates that water is found at even lower latitudes. Future colonists on Mars will be able to tap into these ice deposits, instead of having to travel to much higher latitudes. Another major advantage of LDAs over other sources of Martian water is that they can easily detected and mapped from orbit. Below are examples of lobate debris aprons that were studied with HiRISE.

-
View of lobate debris apron along a slope. Image located in Arcadia quadrangle.
-
Place where a lobate debris apron begins. Note stripes which indicate movement. Image located in Ismenius Lacus quadrangle.
Research, reported in the journal Science in September 2009, demonstrated that some new craters on Mars show exposed, pure, water ice. After a time, the ice disappears, evaporating into the atmosphere. The ice is only a few feet deep. The ice was confirmed with the Compact Imaging Spectrometer (CRISM) on board the Mars Reconnaissance Orbiter (MRO). The ice was found in five locations. Three of the locations are in the Cebrenia quadrangle. These locations are 55.57° N, 150.62° E; 43.28° N, 176.9° E; and 45° N, 164.5° E. Two others are in the Diacria quadrangle: 46.7° N, 176.8° E and 46.33° N, 176.9° E. This discovery proves that future colonists on Mars will be able to obtain water from a wide variety of locations. The ice can be dug up, melted, then taken apart to provide fresh oxygen and hydrogen for rocket fuel. Hydrogen is the powerful fuel used by the space shuttle main engines.
Columnar jointing

In 2009, HiRISE discovered columnar jointing in rocks on Mars. Such jointing is accepted as having involved water. To make the parallel cracks of columnar jointing, more cooling is necessary, and water is the most logical choice. Scientists calculate that the water was present intermittently for a few months to a few years.
Light-toned layered deposits
HiRISE has sent back many images of large surface areas that are termed "light-toned layered deposits." These 30–80 meter thick deposits are believed to have been formed from the action of water. They contain evidence of stream channel systems. Furthermore, chemical data from the Compact Reconnaissance Imaging Spectrometer orbiting the planet have shown water related mineral forms: opal (hydrated silica) and iron sulfates. These can be formed from the action of low temperature acid solutions reacting with basaltic rocks. These features of light-toned layered deposits strongly suggest that there was long lasting precipitation and surface runoff during the Hesperian epoch of Martian history.
Sources of Martian water

Volcanoes give off great amounts of gas when they erupt. The gases are usually water vapor and carbon dioxide. Estimates put the amount of gas released into the atmosphere as enough to make the Martian atmosphere thicker than the Earth's. The water vapor from the volcanoes could have made enough water to place all of Mars under 120 meters of water. In addition, all the carbon dioxide released would have raised the temperature of the planet due to the greenhouse effect, by trapping heat in the form of infrared radiation. So the eruption of lava on Tharsis could have made Mars Earth-like in the past. With a thicker atmosphere, oceans and/or lakes may have been present.
Groundwater on Mars
Main article: Groundwater on MarsOne group of researchers proposed that some of the layers on Mars were caused by groundwater rising to the surface in many places, especially inside of craters. According to the hypothesis, groundwater with dissolved minerals came to the surface, in and later around craters, and helped to form layers by adding minerals (especially sulfate) and cementing sediments. This hypothesis is supported by a groundwater model and by sulfates discovered in a wide area. At first, by examining surface materials with Opportunity Rover, scientists discovered that groundwater had repeatedly risen and deposited sulfates. Later studies with instruments on board the Mars Reconnaissance Orbiter showed that the same kinds of materials exist in a large area that included Arabia.
Evidence of frozen water
Ice patches
On July 28, 2005, the European Space Agency announced the existence of a crater partially filled with frozen water; some then interpreted the discovery as an "ice lake". Images of the crater, taken by the High Resolution Stereo Camera on board the European Space Agency's Mars Express spacecraft, clearly show a broad sheet of ice in the bottom of an unnamed crater located on Vastitas Borealis, a broad plain that covers much of Mars' far northern latitudes, at approximately 70.5° North and 103° East. The crater is 35 km wide and about 2 km deep.
The height difference between the crater floor and the surface of the water ice is about 200 metres. ESA scientists have attributed most of this height difference to sand dunes beneath the water ice, which are partially visible. While scientists do not refer to the patch as a "lake", the water ice patch is remarkable for its size and for being present throughout the year. Deposits of water ice and layers of frost have been found in many different locations on the planet.
Equatorial frozen sea
Surface features consistent with pack ice have been discovered in the southern Elysium Planitia. What appear to be plates of broken ice, ranging in size from 30 m to 30 km, are found in channels leading to a flooded area of approximately the same depth and width as the North Sea. The plates show signs of break up and rotation that clearly distinguish them from lava plates elsewhere on the surface of Mars. The source for the flood is thought to be the nearby geological fault Cerberus Fossae which spewed water as well as lava aged some 2 to 10 million years. It was suggested that the water exited the Cerberus Fossae then pooled and froze in the low, level plains. Not all scientists agree with these conclusions.
Glaciers
Main article: Glaciers on MarsGlaciers formed much of the observable surface in large areas of Mars. Much of the area in high latitudes, especially the Ismenius Lacus quadrangle, is believed to still contain enormous amounts of water ice. Recent evidence has led many planetary scientists to believe that water ice still exists as glaciers with a thin covering of insulating rock. In March 2010, scientists released the results of a radar study of an area called Deuteronilus Mensae that found widespread evidence of ice lying beneath a few meters of rock debris. Glaciers are believed to be associated with Fretted terrain, many volcanoes, and even some craters. Ridges of debris on the surface of the glaciers show the direction of ice movement. The surface of some glaciers has a rough texture due to sublimation of buried ice. The ice goes directly into a gas (this process is called sublimation) and leaves behind an empty space. Overlying material then collapses into the void. Other pictures below show various features that appear to be connected with the existence of glaciers.
-
Gullies and possible remains of old glaciers in a crater in Eridania quadrangle, north of the large crater Kepler. One suspected glacier, to the right, has the shape of a tongue. Image was taken by the Mars Global Surveyor under the Public Target program.
-
Glacier as seen by HiRISE under the HiWish program. Area in rectangle is enlarged in the next photo. Zone of accumulation of snow at the top. Glacier is moving down valley, then spreading out on plain. Evidence for flow comes from the many lines on surface. Location is in Protonilus Mensae in Ismenius Lacus quadrangle.
-
Enlargement of area in rectangle of the previous image. On Earth, the ridge would be called the terminal moraine of an alpine glacier. Picture taken with HiRISE under the HiWish program. Image from Ismenius Lacus quadrangle.
-
Context for the next image of the end of a flow feature or glacier. Location is Hellas quadrangle. Picture taken with HiRISE under the HiWish program.
-
Close-up of the area in the box in the previous image. This may be called by some the terminal moraine of a glacier. For scale, the box shows the approximate size of a football field. Image taken with HiRISE under the HiWish program. Location is Hellas quadrangle.
-
Possible moraine on the end of a past glacier on a mound in Deuteronilus Mensae, as seen by HiRISE, under the HiWish program.
-
Possible Glacial Cirque in Hellas Planitia, as seen by HiRISE, under the HiWish program. Lines are probably due to downhill movement.
-
Glaciers, as seen by HiRISE, under HiWish program. Glacier on left is thin because it has lost much of its ice. Glacier on the right on the other hand is thick; it still contains a lot of ice that is under a thin layer of dirt and rock. Location is Hellas quadrangle.
-
Remains of glaciers, as seen by HiRISE under the HiWish program. Image from Ismenius Lacus quadrangle.
-
Probable glacier as seen by HiRISE under HiWish program. Radar studies have found that it is made up of almost completely pure ice. It appears to be moving from the high ground (a mesa) on the right. Location is Ismenius Lacus quadrangle.
Polar ice caps
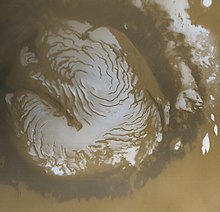
Both the northern polar cap (Planum Boreum) and the southern polar cap (Planum Australe) are believed to grow in thickness during the winter and partially sublime during the summer. Data obtained by the Mars Express satellite made it possible in 2004 to confirm that the southern polar cap has ice at a depth of 3.7 kilometres (2.3 mi) below the surface with varying contents of frozen water, depending on its latitude; the polar cap is a mixture of CO2 ice and water ice. The second part comprises steep slopes known as 'scarps', made almost entirely of water ice, that fall away from the polar cap to the surrounding plains. The third part encompasses the vast permafrost fields that stretch for tens of kilometres away from the scarps. NASA scientists calculate that the volume of water ice in the south polar ice cap, if melted, would be sufficient to cover the entire planetary surface to a depth of 11 metres.
Results, published in 2009, of shallow radar measurements of the North Polar ice cap determined that the volume of water ice in the cap is 821,000 cubic kilometers (197,000 cubic miles). That's equal to 30% of the Earth's Greenland ice sheet or enough to cover the surface of Mars to a depth of 5.6 meters (dividing the ice cap volume by the surface area of Mars is how this number is found). The radar instrument is on board the Mars Reconnaissance Orbiter.
Ground ice
For many years, various scientists have suggested that some Martian surfaces look like periglacial regions on Earth. Sometimes it is said that these are regions of permafrost. These observations suggest that frozen water lies right beneath the surface. A common feature in the higher latitudes, patterned ground, can occur in a number of shapes, including stripes and polygons. On the Earth, these shapes are caused by the freezing and thawing of soil. There are other types of evidence for large amounts of frozen water under the surface of Mars, such as terrain softening which rounds sharp topographical features. Besides landscape features that suggest water frozen in the ground, there is evidence from Mars Odyssey's Gamma Ray Spectrometer, theoretical calculations, and direct measurements with the Phoenix lander.
-
Permafrost polygons in the Arctic
-
Flat terrain near the north pole of Mars showing what appear to be polygonal patterns.
-
Patterned ground in the Canadian Arctic
-
Patterned ground on Mars at 45 degrees north
-
Cones in Athabasca Valles formed from lava interacting with ice.
-
These rings on Mars may have been caused by crust moving over steam produced by lava interacting with water ice.
Some areas of Mars are covered with cones that resemble those on Earth where lava has flowed on top of frozen ground. The heat of the lava melts the ice, then changes it into steam. The powerful force of the steam works its way through the lava and produces a cone. In the Athabasca Valles image above, the larger cones were made when the steam went through the thicker layers of lava. The difference between highest elevation (red) to lowest (dark blue) is 170 metres (560 ft).
Scalloped topography

Certain regions of Mars display scalloped-shaped depressions. The depression are believed to be the remains of an ice-rich mantle deposit. Scallops were caused by ice sublimating from frozen soil. This mantle material probably fell from the air as ice formed on dust when the climate was different due to changes in the tilt of the Mars pole. The scallops are typically tens of meters deep and from a few hundred to a few thousand meters across. They can be almost circular or elongated. Some appear to have coalesced causing a large heavily pitted terrain to form. The process of forming the terrain may begin with sublimation from a crack. There are often polygon cracks where scallops form. So the presence of scalloped topography is an indication of frozen ground.
Possible evidence of flowing water
Main article: Seasonal flows on warm Martian slopesIn August 2011, NASA announced the discovery of seasonal changes in gullies near crater rims on the Southern hemisphere. This suggests salty water flowing and then evaporating, possibly leaving some sort of residue.
On September 27, 2012, NASA scientists announced that the Curiosity rover found evidence for an ancient streambed suggesting a "vigorous flow" of water on Mars. In particular, analysis of an ancient streambed indicated that the water ran quickly, possibly at hip depth. The discovery marks an important achievement for Curiosity, and supports the notion that Mars was once capable of harboring life.
Remnants of the now dried-up stream were found inside the Gale Crater within which Curiosity is working. Proof of running water came in the form of rounded pebbles and gravel fragments that could have only been weathered by strong currents. Their shape and orientation suggests long-distance transport from above the rim of the crater, where a channel named Peace Vallis feeds into the alluvial fan. Because there are many channels like this, NASA scientists believe the flows were continuous or repeated for long durations, and not intermittent.
"From the size of gravels it carried, we can interpret the water was moving about 3 feet per second, with a depth somewhere between ankle and hip deep," noted Curiosity scientist William Dietrich speaking through NASA's official release. "Plenty of papers have been written about channels on Mars with many different hypotheses about the flows in them. This is the first time we're actually seeing water-transported gravel on Mars. This is a transition from speculation about the size of streambed material to direct observation of it."
Mars meteorites

Over thirty meteorites have been found that came from Mars. Some of them contain evidence that they were exposed to water when on Mars.
Some Mars meteorites called basaltic shergottites, appear (from the presence of hydrated carbonates and sulfates) to have been exposed to liquid water prior to injection into space.
It has been shown that another class of meteorites, the nakhlites, were suffused with liquid water around 620 million years ago and that they were ejected from Mars around 10.75 million years ago by an asteroid impact. They fell to Earth within the last 10,000 years.
In 1996, a group of scientists reported on chemical fossils in Allan Hills 84001, a meteorite from Mars. Many studies disputed the validity of the fossils. It was found that most of the organic matter in the meteorite was of terrestrial origin.
Lakes
A variety of lake basins have been discovered on Mars. Some are comparable in size to the largest lakes on Earth, such as the Caspian Sea, Black Sea, and Lake Baikal. Lakes that are fed by valley networks are found in the southern highlands. There are places that are closed depressions with river valleys leading into them. These areas are thought to have once contained lakes. One is in Terra Sirenum which had its overflow move through Ma'adim Vallis into Gusev Crater, which was explored by the Mars Exploration Rover Spirit. Another is near Parana Valles and Loire Vallis. Some lakes are believed to have formed by precipitation, while others were formed from groundwater. Lakes are believed to have existed in the Argyre basin, the Hellas basin, and maybe in Valles Marineris.
Research, published in January 2010, suggests that Mars had lakes, each around 20 km wide, along parts of the equator. Although earlier research showed that Mars had a warm and wet early history that has long since dried up, these lakes existed in the Hesperian Epoch, a much earlier period. Using detailed images from NASA's Mars Reconnaissance Orbiter, the researchers speculate that there may have been increased volcanic activity, meteorite impacts or shifts in Mars' orbit during this period to warm Mars' atmosphere enough to melt the abundant ice present in the ground. Volcanoes would have released gases that thickened the atmosphere for a temporary period, trapping more sunlight and making it warm enough for liquid water to exist. In this new study, channels were discovered that connected lake basins near Ares Vallis. When one lake filled up, its waters overflowed the banks and carved the channels to a lower area where another lake would form. These lakes would be another place to look for evidence of present or past life.
Lake deltas
Researchers have found a number of examples of deltas that formed in Martian lakes. Finding deltas is a major sign that Mars once had a lot of water. Deltas usually require deep water over a long period of time to form. Also, the water level needs to be stable to keep sediment from washing away. Deltas have been found over a wide geographical range and several are pictured below.
-
Delta in Ismenius Lacus quadrangle
-
Delta in Lunae Palus quadrangle
-
Delta in Margaritifer Sinus quadrangle
-
Probable delta in Eberswalde crater
Mars Ocean Hypothesis
The Mars Ocean Hypothesis states that nearly a third of the surface of Mars was covered by an ocean of liquid water early in the planet’s geologic history. This primordial ocean, dubbed Oceanus Borealis, would have filled the Vastitas Borealis basin in the northern hemisphere, a region which lies 4–5 km (2.5–3 miles) below the mean planetary elevation, at a time period of approximately 3.8 billion years ago. Early Mars would require a warmer climate and thicker atmosphere to allow liquid water to remain at the surface.
Observational evidence
There are several physical features in the present geography of Mars that suggest the existence of an ocean. Networks of valleys that merge into larger channels imply erosion by a liquid agent, and resemble ancient riverbeds on Earth. Enormous channels, 25 km wide and several hundred meters deep, appear to direct flow from underground aquifers in the Southern uplands into the Northern plains.
Research published in the Journal of Geophysical Research – Planets, shows a much higher density of flow paths than formerly believed (more than twice as many). Regions on Mars with the most valleys are comparable to what is found on our Earth. In the research, the team developed a computer program to identify valleys by searching for U-shaped structures in topographical data. The large amount of valley networks strongly supports rain on the planet in the past. The global pattern of the martian valleys could be explained with a big northern ocean. A large ocean in the northern hemisphere would explain why there is a southern limit to valley networks; the southernmost regions of Mars, located farthest from the water reservoir, would get little rainfall and would not develop valleys. In a similar fashion the lack of rainfall would explain why Martian valleys become shallower as you go from north to south.
Much of the northern hemisphere of Mars is located at a significantly lower elevation than the rest of the planet (the Martian dichotomy), and is unusually flat. Along the margins of this region are physical features indicative of ancient shorelines. Sea level must follow a line of constant gravitational potential. After adjustment for polar wander caused by mass redistributions from volcanism, the Martian paleo-shorelines meet this criterion. The Mars Orbiter Laser Altimeter (MOLA), which accurately determined the altitude of all parts of Mars, found that the watershed for an ocean on Mars covers three-quarters of the planet.
A study, published in Nature, in June 2010 concluded that an Ocean covered 36% of Mars. The study was based on dozens of deltas that were at the same elevation.
Although phyllosilicates, i.e. clay, have been observed on Mars, the northern lowlands are known to show only few such abundances in early geological layers, a fact that has so far contradicted the theory of a northern ocean on Mars. A 2011 numerical simulation study found though that an ocean of water on the northern hemisphere would have had a temperature near the freezing point "which would have hindered the formation of phyllosilicate minerals in the ocean basin" and would therefore explain the relative absence of clay minerals on the northern hemisphere.
Theoretical issues
The existence of liquid water on the surface of Mars requires both a warmer and thicker atmosphere. Atmospheric pressure on the present day Martian surface only exceeds that of the triple point of water (6.11 hPa) in the lowest elevations; at higher elevations water can exist only in solid or vapor form. Annual mean temperatures at the surface are currently less than 210 K, significantly less than what is needed to sustain liquid water. However, early in its history Mars may have had conditions more conducive to retaining liquid water at the surface.
Calculations of the volume of one of the supposed oceans yielded a number that would mean that Mars was covered with as much water as the Earth.
The water that was in this ocean may have escaped into space, been deposited in the ice caps, or have been trapped in the soil.
Alternative ideas
The existence of a primordial Martian ocean remains controversial among scientists. The Mars Reconnaissance Orbiter's High Resolution Imaging Science Experiment has discovered large boulders on the site of the ancient seabed, which should contain only fine sediment. The interpretations of some features as ancient shorelines has been challenged. Some have been shown to be of volcanic origin.
Possibility of Mars having enough water to support life
Life is generally understood to require liquid water. Some evidence suggests that Mars had enough water to form lakes and to carve huge river valleys. Vast quantities of water have been discovered frozen beneath much of the Martian surface. Nevertheless, many significant issues remain.
- History. When did the water once flow on Mars? Mars areas have been extremely dry for long periods, as marked by the presence of olivine that would be decomposed by water. On the other hand, many other areas contain clay and/or sulfates, which indicate the presence of liquid water on the surface.
- Sulfates. While the presence of sulfates bolsters the case for surface water, they present problems of their own. Sulfates form under acid conditions. On Earth some organisms can survive in acidic environment, but questions remain about the possibility of life forming under such conditions. Even allowing for adaptation to acidic environments, could life actually originate in acidic waters? On the other hand, carbonates, which do not form in acid solutions, have been found in Martian meteorites by the Phoenix lander and by the Compact Reconnaissance Imaging Spectrometer, an instrument aboard the NASA Mars Reconnaissance Orbiter.
- Salts. The saltiness of the soil could be a major obstacle for life. Salt has been used by the human race as a major preservative since most organisms can not live in highly salted water (halophile bacteria being an exception).
- Oxidizers. The Phoenix mission discovered perchlorate, a highly oxidizing chemical in the soil. Although some organisms use perchlorate, the chemical could be hostile to life. Other research show that there is a variety of soil types on Mars including clays and alkaline soil as well as acidic soil, and studies of Mars analog soils find that they are not unusually or severely biotoxic, and not growth limiting for Mars microbiota (if present).
Benton Clark III, a member of the Mars Exploration Rover (MER) team, surmises that Martian organisms could be adapted to a sort of suspended animation for millions of years. Indeed, some organisms can endure extreme environments for a time. Measurements performed on Earth under 50 meters of permafrost, showed that half of the microorganisms would accumulate enough radiation from radioactive decay in rocks to die in 10 million years, but if organisms come back to life every few million years they could repair themselves and reset any damaged systems, especially DNA. Other scientists are in agreement.
The discovery of organisms living in extreme conditions on Earth has brought renewed hope that life exists, or once existed on Mars. Colonies of microbes have been found beneath almost 3 kilometers of glaciers in the Canadian Arctic and in Antarctica. Could microbes live under the ice caps of Mars? In the 1980s, it was thought that microorganisms might live up to a depth of a few meters under ground. Today, we know that a wide variety of organisms grow to a depth of over a mile. Some live on gases like methane, hydrogen, and hydrogen sulfide that originate from volcanic activity. Mars has had widespread volcanic activity. It is entirely possible that life exists near volcanoes or underground reservoirs of hot magma. Some organisms live inside of basalt (the most common rock on Mars) and produce methane. Methane has been tracked on Mars. Some believe there must be some (possibly biological) mechanism that is producing methane since it will not last long in the present atmosphere of Mars. Other organisms eat sulfur compounds; the same chemicals that have been found in many regions of Mars. Scientists have suggested that whole communities of organisms could thrive near areas heated by volcanic activity. Studies have shown that certain forms of life have adapted to extremely high temperatures (80° to 110 °C). With all the volcanic activity on Mars, one would suppose that certain places have not yet cooled down. An underground magma chamber might melt ice, then circulate water through the ground. Remains of hot springs like the ones in Yellowstone National Park have actually been spotted by the Mars Reconnaissance Orbiter. Minerals associated with hot springs, such as opal and silica have been studied on the ground by Spirit Rover and mapped from orbit by the Mars Reconnaissance Orbiter. Some volcanoes, like Olympus Mons, seem relatively young to the eyes of a geologist. However, no warm areas have ever been found on the surface. The Mars Global Surveyor scanned most of the surface in infrared with its TES instrument. The Mars Odyssey's THEMIS, also imaged the surface in wavelengths that measure temperature.
The possibility of liquid water on Mars has been examined. Although water would quickly boil or evaporate away, lake-sized bodies of water would quickly be covered with an ice layer which would greatly reduce evaporation. With a cover of dust and other debris, water under ice might last for some time and could even flow to significant distances as ice-covered rivers. Lake Vostok in Antarctica may have implications for liquid water still being on Mars because if the lake existed before the perennial glaciation began, is likely that the lake did not freeze all the way to the bottom. Accordingly if water existed before the polar ice caps on Mars, it is likely that there is still liquid water below the ice caps. Large quantities of water could be released, even today, by an asteroid impact. It has been suggested that life has survived over millions of years by periodic impacts which melted ice and allowed organisms to come out of dormancy and live for a few thousands of years. But if impacts brought the water, maybe liquid water did not exist on the surface very long. Large river valleys could have been made in short periods of time (maybe just days) when impacts caused water to flow as a giant flood. We suppose that Mars had great amounts of water because of the existence of so many large river valleys. Maybe, valleys did not take thousands to millions of years to form as on the Earth. It is accepted that a vast network of channels, resembling many Martian channels, were formed in a very short time period in eastern Washington State when floods were caused by a breakout of an ice-dammed lake. So, perhaps not that much water was involved and maybe it did not last long enough for life to develop.
Studies have shown that various salts present in the Martian soil could act as a kind of antifreeze—keeping water liquid well below its normal freezing point. Some calculations suggest that tiny amounts of liquid water may be present for short periods of time (hours) in some locations. Some researchers have calculated that when taking into consideration insolation and pressure factors that liquid water could exist in some areas for about 10% of the Martian year; others estimate that water could be a liquid for only 2% of the year. Either way, that may be enough liquid water to support some forms of hardy organisms. It may not take much liquid water for life; organisms have been found on Earth living on extremely thin layers of unfrozen water in below-freezing locations. Research described in December 2009, showed that liquid water could form in the daytime inside of snow on Mars. As light heats ice, it may be warming up dust grains located inside. These grains would then store heat and form water by melting some of the ice. The process has been already been observed in Antarctica. Enough water may be produced for physical, chemical, and biological processes..
Another location under consideration is in underground caves on Mars. There is some evidence for possible subsurface ice sheets near the equator. This may for instance be geologically ancient ice which may melt or sublimate on its way towards the surface.
Experiments also suggest that lichen and bacteria may also be able to survive solely on humidity from the air, particularly in cracks in the rocks. The water is present in the morning and evening when humidity briefly condenses as precipitation across the surface, and the organisms can absorb it.
Valleys and channels
Further information: Atmosphere of Mars
The Viking Orbiters caused a revolution in our ideas about water on Mars. Huge river valleys were found in many areas. They showed that floods of water broke through dams, carved deep valleys, eroded grooves into bedrock, and traveled thousands of kilometers. Areas of branched streams, in the southern hemisphere, suggested that rain once fell.
The images below, some of the best from the Viking Orbiters, are mosaics of many small, high resolution images. Click on the images for more detail. Some of the pictures are labeled with place names.
-
Streamlined Islands seen by Viking showed that large floods occurred on Mars. Image is located in Lunae Palus quadrangle.
-
Tear-drop shaped islands caused by flood waters from Maja Vallis, as seen by Viking Orbiter. Image is located in Oxia Palus quadrangle. The islands are formed in the ejecta of Lod Crater, Bok Crater, and Gold Crater.
-
Scour Patterns, located in Lunae Palus quadrangle, were produced by flowing water from Maja Vallis, which lies just to the left of this mosaic. Detail of flow around Dromore Crater is shown on the next image.
-
Great amounts of water were required to carry out the erosion shown in this Viking image. Image is located in Lunae Palus quadrangle. The erosion shaped the ejecta around Dromore Crater.
-
Waters from Vedra Vallis, Maumee Vallis, and Maja Valles went from Lunae Planum on the left, to Chryse Planitia on the right. Image is located in Lunae Palus quadrangle and was taken by Viking Orbiter.
-
Area around Northern Kasei Valles, showing relationships among Kasei Valles, Bahram Vallis, Vedra Vallis, Maumee Vallis, and Maja Valles. Map location is in Lunae Palus quadrangle and includes parts of Lunae Planum and Chryse Planitia.
-
The ejecta from Arandas Crater acts like mud. It moves around small craters (indicated by arrows), instead of just falling down on them. Craters like this suggest that large amounts of frozen water were melted when the impact crater was produced. Image is located in Mare Acidalium quadrangle and was taken by Viking Orbiter.
-
This view of the flank of Alba Patera shows several chnnels/troughs. Some channels are associated with lava flows; others are probably caused by running water. A large trough or graben turns into a line of collapse pits. Image is located in Arcadia quadrangle and was taken by Viking Orbiter.
-
Branched channels in Thaumasia quadrangle, as seen by Viking Orbiter. Networks of channels like this are strong evidence for rain on Mars in the past.
-
The branched channels seen by Viking from orbit strongly suggested that it rained on Mars in the past. Image is located in Margaritifer Sinus quadrangle.
-
Ravi Vallis, as seen by Viking Orbiter. Ravi Vallis was probably formed when catastrophic floods came out of the ground to the right (chaotic terrain). Image located in Margaritifer Sinus quadrangle.
-
Channels near Warrego Valles. These branched channels are strong evidence for flowing water on Mars, perhaps during a much warmer period.
-
Semeykin Crater Drainage. Click on image to see details of drainage system. Location is Ismenius Lacus quadrangle.
-
Channels in Candor plateau, as seen by HiRISE. Location is Coprates quadrangle. Click on image to see many small, branched channels which are strong evidence for sustained precipitation.
-
Channels near the rim of Ius Chasma, as seen by HiRISE. The pattern and high density of these channels support precipitation as the source of the water. Location is Coprates quadrangle.
-
Stream meander and cutoff, as seen by HiRISE under HiWish program. This image is located in Mare Acidalium quadrangle.
The high resolution Mars Orbiter Camera on the Mars Global Surveyor has taken pictures which give much more detail about the history of liquid water on the surface of Mars. Despite the many gigantic flood channels and associated tree-like network of tributaries found on Mars, there are no smaller scale structures that would indicate the origin of the flood waters. It has been suggested that weathering processes have denuded these, indicating the river valleys are old features. Another theory about the formation of the ancient river valleys is that rather than floods, they were created by the slow seeping out of groundwater. This observation is supported by the sudden ending of the river networks in theatre shaped heads, rather than tapering ones. Additionally, valleys are often discontinuous, small sections of uneroded land separating the parts of the river.
On the other hand, evidence in favor of heavy or even catastrophic flooding is found in the giant ripples in the Athabasca Vallis.
Research, published in the Journal of Geophysical Research in June 2010, reported the detection of 40,000 river valleys on Mars, about four times the number of river valleys that have previously been identified by scientists.
Many Mars researchers now agree that the Martian water worn features can be divided into two distinct classes: 1. dendritic (branched), terrestrial-scale, widely distributed, Noachian-age "valley networks" and 2. exceptionally large, long, single-thread, isolated, uncommon, Hesperian-age "outflow channels". Consensus seems to be emerging that the latter formed in single, catastrophic ruptures of subsurface water reservoirs, possibly sealed by ice, discharging colossal quantities of water across an otherwise ultra-arid Mars surface. The former, however, probably indicate prolonged "wet" (though still arid by terrestrial standards) conditions on Noachian-era Mars, with an active ongoing hydrological cycle.
Higher resolution observations from spacecraft like Mars Global Surveyor also revealed at least a few hundred features along crater and canyon walls that appear similar to terrestrial seepage gullies. The gullies tended to be Equator facing and in the highlands of the southern hemisphere, and all greater than 30° north or south latitude. The researchers found no partially degraded (i.e. weathered) gullies and no superimposed impact craters, indicating that these are very young features.
-
Group of gullies on north wall of crater that lies west of the crater Newton (41.3047 degrees south latitude, 192.89 east longitide). Image taken with Mars Global Surveyor under the public target program.
-
Crater wall inside Mariner Crater showing a large group of gullies, as seen by HiRISE.
-
Gullies with branches.
-
Group of deep gullies, as seen by HiRISE.
Liquid water
Liquid water cannot exist on the surface of Mars with its present low atmospheric pressure, except at the lowest elevations for short periods. Recently, the discovery of gully deposits that were not seen ten years ago provided evidence to support the popular belief that liquid water flowed on the surface in the recent past. There is some disagreement in the scientific community as to whether or not the new gully deposits were formed from liquid water. A paper, published in the January 2010 issue of Icarus, concluded that the observed deposits were probably dry flows that were started by a rockfall in steep regions.
Among the findings from the Opportunity rover is the presence of hematite on Mars in the form of small spheres on the Meridiani Planum. The spheres are only a few millimetres in diameter and are believed to have formed as rock deposits under watery conditions billions of years ago. Other minerals have also been found containing forms of sulfur, iron, or bromine such as jarosite. This and other evidence led a group of 50 scientists to conclude in the December 9, 2004 edition of the journal Science that "Liquid water was once intermittently present at the Martian surface at Meridiani, and at times it saturated the subsurface. Because liquid water is a key prerequisite for life, we infer conditions at Meridiani may have been habitable for some period of time in Martian history." Later studies suggested that this liquid water was actually acid because of the types of minerals found at the location. On the opposite side of the planet, the mineral goethite, which (unlike hematite) forms only in the presence of water, along with other evidence of water, has also been found by the Spirit rover in the "Columbia Hills".
On July 31, 2008, NASA announced that the Phoenix lander confirmed the presence of water ice on Mars, as predicted on 2002 by the Mars Odyssey orbiter.
Studies have shown that various salts present in the Martian soil could act as a kind of antifreeze—keeping water liquid at temperatures far below its normal freezing point. Some calculations suggest that tiny amounts of liquid water may be present for short periods of time (hours) in some locations. Some researchers have calculated that when taking into consideration insolation and pressure factors that liquid water could exist in some areas for about 10% of the Martian year; others estimate that water could be a liquid for only 2% of the year. Either way, that may be enough liquid water to support some forms of hardy organisms. It may not take much liquid water for life—organisms have been found on Earth living on extremely thin layers of unfrozen water in below-freezing locations. Research in December 2009 showed that liquid water could form in the daytime inside of snow on Mars. As light heats ice, it may be warming up dust grains located inside. These grains would then store heat and form water by melting some of the ice. This process has already been observed in Antarctica. Enough water may be produced for physical, chemical, and biological processes.
Polar ice caps
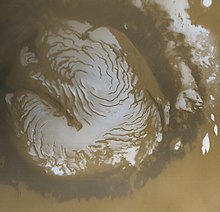

Both the northern polar cap (Planum Boreum) and the southern polar cap (Planum Australe) are believed to grow in thickness during the winter and partially sublime during the summer. Data obtained by the Mars Express satellite, made it possible in 2004 to confirm that the southern polar cap has ice at a depth of 3.7 kilometres (2.3 mi) below the surface with varying contents of frozen water depending on its latitude. The polar cap is a mixture of CO2 ice and water ice. The second part comprises steep slopes known as scarps, made almost entirely of water ice, that fall away from the polar cap to the surrounding plains. The third part encompasses the vast permafrost fields that stretch for tens of kilometres away from the scarps. NASA scientists calculate that the volume of water ice in the south polar ice cap, if melted, would be sufficient to cover the entire planetary surface to a depth of 11 metres.
Research, published in January 2010 using HiRISE images, says that understanding the layers is more complicated than was formerly believed. The brightness of the layers does not just depend on the amount of dust. The angle of the sun together with the angle of the spacecraft greatly affect the brightness seen by the camera. This angle depends on factors such as the shape of the trough wall and its orientation. Furthermore, the roughness of the surface can greatly change the albedo (amount of reflected light). In addition, many times what one is seeing is not a real layer, but a fresh covering of frost. All of these factors are influenced by the wind which can erode surfaces. The HiRISE camera did not reveal layers that were thinner than those seen by the Mars Global Surveyor. However, it did see more detail within layers.
Ice patches
On July 28, 2005, the European Space Agency announced the existence of a crater partially filled with frozen water, which some then interpreted as an "ice lake". Images of the crater, taken by the High Resolution Stereo Camera on board the European Space Agency's Mars Express spacecraft, clearly show a broad sheet of ice in the bottom of an unnamed crater located on Vastitas Borealis, a broad plain that covers much of Mars' far northern latitudes, at approximately 70.5° North and 103° East. The crater is 35 km wide and about 2 km deep.
The height difference between the crater floor and the surface of the water ice is about 200 metres. ESA scientists have attributed most of this height difference to sand dunes beneath the water ice, which are partially visible. While scientists do not refer to the patch as a "lake", the water ice patch is remarkable for its size and for being present throughout the year. Deposits of water ice and layers of frost have been found in many different locations on the planet.
Equatorial frozen sea
Surface features consistent with pack ice have been discovered in the southern Elysium Planitia. What appear to be plates of broken ice, ranging in size from 30 m to 30 km, are found in channels leading to a flooded area of approximately the same depth and width as the North Sea. The plates show signs of break up and rotation that clearly distinguish them from lava plates elsewhere on the surface of Mars. The source for the flood is thought to be the nearby geological fault, Cerberus Fossae, which spewed water as well as lava some 2 to 10 million years ago.
Ancient coastline
A striking feature of the topography of Mars is the flat plains of the northern hemisphere. With the increasing amounts of data returning from the current set of orbiting probes, what seems to be an ancient shoreline several thousands of kilometres long has been discovered. Actually, two different shorelines have been proposed. One, the Arabia shoreline, can be traced all around Mars except through the Tharsis volcanic region. The second, the Deuteronilus, follows the Vastitas Borealis Formation. Some researchers do not agree that these formations are real shorelines. One major problem with the conjectured 2 Ga old shoreline is that it is not flat — i.e. does not follow a line of constant gravitational potential. However, a 2007 Nature article points out that this could be due to a change in distribution in Mars' mass, perhaps due to volcanic eruption or meteor impact—the Elysium volcanic province or the massive Utopia basin that is buried beneath the northern plains have been put forward as the most likely causes. The Mars Ocean Hypothesis conjectures that the Vastitas Borealis basin was the site of a primordial ocean of liquid water 3.8 billion years ago.
Glaciers and ice ages

Many large areas of Mars have been shaped by glaciers. Much of the area in high latitudes, especially the Ismenius Lacus quadrangle, are believed to still contain enormous amounts of water ice. Recent evidence has led many planetary scientists to believe that water ice still exists as glaciers with thin coverings of insulating rock. In March 2010, scientists released the results of a radar study of an area called Deuteronilus Mensae that found widespread evidence of ice lying beneath a few meters of rock debris. Glaciers are believed to be associated with Fretted terrain, many volcanoes, and even some craters. Researchers have described glacial deposits on Hecates Tholus, Arsia Mons, Pavonis Mons, and Olympus Mons.
Ridges of debris on the surface of the glaciers indicate the direction of ice movement. The surface of some glaciers have rough textures due to sublimation of buried ice. The ice goes directly into a gas (this process is called sublimation) and leaves behind an empty space. Overlying material then collapses into the void. Glaciers are not pure ice; they contain dirt and rocks. At times, they dump their loads of material into ridges. Such ridges are called moraines. Some places on Mars have groups of ridges that are twisted around; this may have been due to more movement after the ridges were put into place. Sometimes chunks of ice fall from the glacier and get buried in the land surface. When they melt, a more or less round hole remains. On Earth we call these features kettles or kettle holes. Mendon Ponds Park in upstate NY has preserved several of these kettles. The picture from HiRISE below shows possible kettles in Moreux Crater.
Pictures below show various features that appear to be connected with the existence of glaciers.
-
Moreux Crater moraines and kettle holes, as seen by HIRISE. Location is Ismenius Lacus quadrangle.
-
Niger Vallis with features typical of this latitude. Chevron pattern results from movement of ice-rich material. Click on image to see chevron pattern and mantle. Location is Hellas quadrangle.
-
Mesa in Ismenius Lacus quadrangle, as seen by CTX. Mesa has several glaciers eroding it. One of the glaciers is seen in greater detail in the next two images from HiRISE.
-
Glacier as seen by HiRISE under the HiWish program. Area in rectangle is enlarged in the next photo. Zone of accumulation of snow at the top. Glacier is moving down valley, then spreading out on plain. Evidence for flow comes from the many lines on surface. Location is in Protonilus Mensae in Ismenius Lacus quadrangle.
-
Enlargement of area in rectangle of the previous image. On Earth the ridge would be called the terminal moraine of an alpine glacier. Picture taken with HiRISE under the HiWish program.
Many mid-latitude craters contain straight and/or curved ridges of material that resemble glacial moraines on the Earth. Moving ice carries rock material, then drops it as the ice disappears. On Mars, with its extremely thin atmosphere, ice does not usually melt but instead sublimates. As a result, the rock debris is just dropped, and melt water is not produced so the remains of these glaciers do not appear the same as on the Earth. Various names have been applied to these ridged features. Depending on the author, they may be called arcuate ridges, viscous flow features, Martian flow features, or moraine-like ridges. Many, but not all, seem to be associated with gullies on the walls of craters and mantling material.
-
Gullies and possible remains of old glaciers in a crater in Eridania quadrangle, north of the large crater Kepler. One suspected glacier, to the right, has the shape of a tongue. Image taken with Mars Global Surveyor, under the Public Target program.
-
Tongue-Shaped Glacier, as seen by Mars Global Surveyor. Location is Hellas quadrangle.
-
Tongue-shaped glacier, as seen by HiRISE under the HiWish program. Ice may exist in the glacier, even today, beneath an insulating layer of dirt. Location is Hellas quadrangle.
-
Close-up of tongue-shaped glacier, as seen by HiRISE under the HiWish program. Resolution is about 1 meter, so one can see objects a few meters across in this image. Ice may exist in the glacier, even today, beneath an insulating layer of dirt. Location is Hellas quadrangle.
Lineated deposits are probably rock-covered glaciers which are found on the floors of some channels. Their surfaces have ridged and grooved materials that deflect around obstacles, similar to some glaciers on the Earth. Lineated floor deposits may be related to Lobate Debris Aprons, which have been proven to contain large amounts of ice by orbiting radar.
For many years, researchers believed that on Mars features called Lobate Debris Aprons looked like glacial flows. It was thought that ice existed under a layer of insulating rocks. With new instrument readings, it has been confirmed that Lobate Debris Aprons contain almost pure ice that is covered with a layer of rocks.
- Reull Vallis with lineated floor deposits. Click on image to see relationship to other features. Floor deposits are believed to be formed from ice movement. Location is Hellas quadrangle. Reull Vallis with lineated floor deposits. Click on image to see relationship to other features. Floor deposits are believed to be formed from ice movement. Location is Hellas quadrangle.
-
Coloe Fossae Lineated Valley Fill, as seen by HiRISE. Scale bar is 500 meters long. Location is Ismenius Lacus quadrangle.
Ice ages on Mars are far different than the ones that our Earth experiences. Ice ages on Mars, that is when ice accumulates, occur during warmer periods. During a Martian ice age, the poles get warmer. Water ice leaves the ice caps and is deposited in mid latitudes. The moisture from the ice caps travels to lower latitudes in the form of deposits of frost or snow mixed generously with dust. The atmosphere of Mars contains a great deal of fine dust particles. Water vapor condenses on these particles, which then fall down to the ground due to the additional weight of the water coating. When ice at the top of the mantling layer returns to the atmosphere, it leaves behind dust which serves to insulate the remaining ice. The total volume of water removed is about a few percent of the ice caps, or enough to cover the entire surface of the planet under one meter of water. Much of this moisture from the ice caps results in a thick smooth mantle that is thought to be a mixture of ice and dust. This ice-rich mantle, a few yards thick, smoothes the land. But in places it displays a bumpy texture, resembling the surface of a basketball. Because there are few craters on this mantle, the mantle is relatively young. It is believed that this mantle was put in place during a relatively recent ice age. The mantle covers areas to the equivalent latitude of Saudi Arabia and the southern United States.
The images below, all taken with HiRISE show a variety of views of this smooth mantle.
-
Ptolemaeus Crater Rim. Click on image to see excellent view of mantle deposit. Location is Phaethontis quadrangle.
-
Atlantis Chaos. Click on image to see mantle covering and possible gullies. The two images are different parts of the original image. They have different scales. Location is Phaethontis quadrangle.
-
Dissected mantle with layers. Location is Noachis quadrangle.
Ice ages are driven by changes in Mars's orbit and tilt. Orbital calculations show that Mars wobbles on its axis far more than Earth. Earth is stabilized by its proportionally large moon, so it only wobbles a few degrees. Mars, in contrast, may change its tilt by tens of degrees. Its poles get much more direct sunlight at times, which causes the ice caps to warm and become smaller as ice sublimes. Adding to the variability of the climate, the eccentricity of the orbit of Mars changes twice as much as Earth's eccentricity. Computer simulations have shown that a 45° tilt of the Martian axis would result in ice accumulation in areas that display glacial landforms. A 2008 study provided evidence for multiple glacial phases during Late Amazonian glaciation at the dichotomy boundary on Mars.
Glaciers on volcanoes
Using new MGS and Odyssey data, combined with recent developments in the study of cold-based glaciers, scientists believe glaciers once existed and still exist on some volcanoes. The evidence for this are concentric ridges (these are moraines dropped by the glacier), a knobby area (caused by ice sublimating), and a smooth section that flows over other deposits (debris-covered glacial ice). The ice could have been deposited when the tilt of Mars changed the climate, thereby causing more moisture to be present in the atmosphere. Studies suggest the glaciation happened in the Late Amazonian period, the latest period in Mars history. Multiple stages of glaciations probably occurred. The ice present today represents one more resource for the possible future colonization of the planet. Researchers have described glacial deposits on Hecates Tholus, Arisia Mons, Pavonis Mons, and Olympus Mons.
See also
References
- "Mars Global Surveyor Measures Water Clouds". Retrieved March 7, 2009.
- "Flashback: Water on Mars Announced 10 Years Ago". SPACE.com. June 22, 2000. Retrieved December 19, 2010.
- "Science@NASA, The Case of the Missing Mars Water". Retrieved March 7, 2009.
- ISBN 0-312-24551-3
- "PSRD: Ancient Floodwaters and Seas on Mars". Psrd.hawaii.edu. July 16, 2003. Retrieved December 19, 2010.
- "Gamma-Ray Evidence Suggests Ancient Mars Had Oceans | SpaceRef – Your Space Reference". SpaceRef. November 17, 2008. Retrieved December 19, 2010.
- ^ Carr, M.; Head, J. (2003). "Oceans on Mars: An assessment of the observational evidence and possible fate". Journal of Geophysical Research. 108: 5042. Bibcode:2003JGRE..108.5042C. doi:10.1029/2002JE001963.
{{cite journal}}
: Cite has empty unknown parameter:|author-name-separator=
(help); Unknown parameter|author-separator=
ignored (help) - Harwood, William (January 25, 2013). "Opportunity rover moves into 10th year of Mars operations". Space Flight Now. Retrieved February 18, 2013.
- "Water at Martian south pole". March 17, 2004. Retrieved September 29, 2009.
- ^ "ch4". History.nasa.gov. Retrieved December 19, 2010.
- ^ Harrison, K; Grimm, R. (2005). "Groundwater-controlled valley networks and the decline of surface runoff on early Mars". Journal of Geophysical Research. 110. Bibcode:2005JGRE..11012S16H. doi:10.1029/2005JE002455.
{{cite journal}}
: Cite has empty unknown parameter:|author-name-separator=
(help); Unknown parameter|author-separator=
ignored (help) - ^ Howard, A.; Moore, Jeffrey M.; Irwin, Rossman P. (2005). "An intense terminal epoch of widespread fluvial activity on early Mars: 1. Valley network incision and associated deposits". Journal of Geophysical Research. 110. Bibcode:2005JGRE..11012S14H. doi:10.1029/2005JE002459.
- ^ Hugh H. Kieffer (1992). Mars. University of Arizona Press. ISBN 978-0-8165-1257-7. Retrieved March 7, 2011.
- "New Signs That Ancient Mars Was Wet". Space.com. October 28, 2008. Retrieved February 10, 2013.
- ^ "Articles | Was there life on Mars? – ITV News". Itv.com. Retrieved December 19, 2010.
- Glotch, T. and P. Christensen. 2005. Geologic and mineralogical mapping of Aram Chaos: Evidence for water-rich history. J. Geophys. Res. 110. doi:10.1029/2004JE002389
- ^ Irwin, Rossman P.; Howard, Alan D.; Craddock, Robert A.; Moore, Jeffrey M. (2005). "An intense terminal epoch of widespread fluvial activity on early Mars: 2. Increased runoff and paleolake development". Journal of Geophysical Research. 110. Bibcode:2005JGRE..11012S15I. doi:10.1029/2005JE002460.
- ^ Fassett, C.; Head, III (2008). "Valley network-fed, open-basin lakes on Mars: Distribution and implications for Noachian surface and subsurface hydrology". Icarus. 198: 37–56. Bibcode:2008Icar..198...37F. doi:10.1016/j.icarus.2008.06.016.
{{cite journal}}
: Cite has empty unknown parameter:|author-name-separator=
(help); Unknown parameter|author-separator=
ignored (help) - ^ Parker, T.; Clifford, S. M.; Banerdt, W. B. (2000). "Argyre Planitia and the Mars Global Hydrologic Cycle" (PDF). Lunar and Planetary Science. XXXI: 2033. Bibcode:2000LPI....31.2033P.
- ^ Heisinger, H.; Head, J. (2002). "Topography and morphology of the Argyre basin, Mars: implications for its geologic and hydrologic history". Planet. Space Sci. 50 (10–11): 939–981. Bibcode:2002P&SS...50..939H. doi:10.1016/S0032-0633(02)00054-5.
{{cite journal}}
: Cite has empty unknown parameter:|author-name-separator=
(help); Unknown parameter|author-separator=
ignored (help) - ^ ISBN 978-0-521-87201-0
- ^ Moore, J.; Wilhelms, D. (2001). "Hellas as a possible site of ancient ice-covered lakes on Mars". Icarus. 154 (2): 258–276. Bibcode:2001Icar..154..258M. doi:10.1006/icar.2001.6736.
{{cite journal}}
: Cite has empty unknown parameter:|author-name-separator=
(help); Unknown parameter|author-separator=
ignored (help) - ^ Weitz, C.; Parker, T. (2000). "New evidence that the Valles Marineris interior deposits formed in standing bodies of water" (PDF). Lunar and Planetary Science. XXXI: 1693. Bibcode:2000LPI....31.1693W.
- ^ Morton, O. 2002. Mapping Mars. Picador, NY, NY
- Head, JW; Neukum, G; Jaumann, R; Hiesinger, H; Hauber, E; Carr, M; Masson, P; Foing, B; Hoffmann, H (2005). "Tropical to mid-latitude snow and ice accumulation, flow and glaciation on Mars". Nature. 434 (7031): 346–350. Bibcode:2005Natur.434..346H. doi:10.1038/nature03359. PMID 15772652.
- Head, J. and D. Marchant. 2006. Evidence for global-scale northern mid-latitude glaciation in the Amazonian period of Mars: Debris-covered glacial and valley glacial deposits in the 30 - 50 N latitude band. Lunar. Planet. Sci. 37. Abstract 1127
- Head, J. and D. Marchant. 2006. Modifications of the walls of a Noachian crater in Northern Arabia Terra (24 E, 39 N) during northern mid-latitude Amazonian glacial epochs on Mars: Nature and evolution of Lobate Debris Aprons and their relationships to lineated valley fill and glacial systems. Lunar. Planet. Sci. 37. Abstract 1128
- Head, J., et al. 2006. Modification if the dichotomy boundary on Mars by Amazonian mid-latitude regional glaciation. Geophys. Res Lett. 33
- Garvin, J. et al. 2002. Lunar Planet. Sci: 33. Abstract # 1255.
- ^ "Mars Odyssey: Newsroom". Mars.jpl.nasa.gov. May 28, 2002. Retrieved December 19, 2010.
- ^ Feldman, W. C. (2004). "Global distribution of near-surface hydrogen on Mars". J. Geographical Research. 109. Bibcode:2004JGRE..10909006F. doi:10.1029/2003JE002160.
- "Radar evidence for ice in lobate debris aprons in the mid-northern latitudes of Mars" (PDF). Planetary.brown.edu. Retrieved February 10, 2013.
- Head, J. et al. 2005. Tropical to mid-latitude snow and ice accumulation, flow and glaciation on Mars. Nature: 434. 346-350
- Source: Brown University Posted Monday, October 17, 2005 (October 17, 2005). "Mars' climate in flux: Mid-latitude glaciers | SpaceRef - Your Space Reference". Marstoday.com. Retrieved February 10, 2013.
{{cite web}}
: CS1 maint: multiple names: authors list (link) CS1 maint: numeric names: authors list (link) - (April 23, 2008). "Glaciers Reveal Martian Climate Has Been Recently Active | Brown University News and Events". News.brown.edu. Retrieved February 10, 2013.
{{cite web}}
: Text " Contact: Richard Lewis " ignored (help); Text " " ignored (help)CS1 maint: extra punctuation (link) - Plaut, J. et al. 2008. Radar Evidence for Ice in Lobate Debris Aprons in the Mid-Northern Latitudes of Mars. Lunar and Planetary Science XXXIX. 2290.pdf
- Holt, J. et al. 2008. Radar Sounding Evidence for Ice within Lobate Debris Aprons near Hellas Basin, Mid-Southern Latitudes of Mars. Lunar and Planetary Science XXXIX. 2441.pdf
- ^ Bright Chunks at Phoenix Lander's Mars Site Must Have Been Ice – Official NASA press release (June 19, 2008)
- ^ Rayl, A. j. s. (June 21, 2008). "Phoenix Scientists Confirm Water-Ice on Mars". The Planetary Society web site. Planetary Society. Retrieved June 23, 2008.
{{cite web}}
: External link in
(help)|work=
- ^ "Confirmation of Water on Mars". Nasa.gov. June 20, 2008. Retrieved December 19, 2010.
- ^ Witeway, J.; Komguem, L; Dickinson, C; Cook, C; Illnicki, M; Seabrook, J; Popovici, V; Duck, TJ; Davy, R (2009). "Mars Water-Ice Clouds and Precipation". Science. 325 (5936): 68–70. Bibcode:2009Sci...325...68W. doi:10.1126/science.1172344. PMID 19574386.
- ^ "Liquid Saltwater Is Likely Present On Mars, New Analysis Shows". Sciencedaily.com. March 20, 2009. Retrieved August 20, 2011.
- ^ ISBN 978-1-60598-176-5
- ^ Rennó, Nilton O.; Bos, Brent J.; Catling, David; Clark, Benton C.; Drube, Line; Fisher, David; Goetz, Walter; Hviid, Stubbe F.; Keller, Horst Uwe (2009). "Possible physical and thermodynamical evidence for liquid water at the Phoenix landing site". Journal of Geophysical Research. 114. Bibcode:2009JGRC..11400E03R. doi:10.1029/2009JE003362.
- Staff (July 2, 2012). "Ancient Mars Water Existed Deep Underground". Space.com. Retrieved July 3, 2012.
- Forget, F., et al. 2006. Planet Mars Story of Another World. Praxis Publishing, Chichester, UK. ISBN 978-0-387-48925-4
- Carr, M. 2006. The Surface of Mars. Cambridge University Press. ISBN 978-0-521-87201-0
- Craddock, R.; Howard, A. (2002). "The case for rainfall on a warm, wet early Mars". J. Geophys. Res. 107: E11.
- Head, J.; et al. (2006). "Extensive valley glacier deposits in the northern mid-latitudes of Mars: Evidence for the late Amazonian obliquity-driven climate change". Earth Planet. Sci. Lett. 241: 663–671.
{{cite journal}}
: Explicit use of et al. in:|first1=
(help) - Madeleine, J. et al. 2007. Mars: A proposed climatic scenario for northern mid-latitude glaciation. Lunar Planet. Sci. 38. Abstract 1778.
- Madeleine, J.; et al. (2009). "Amazonian northern mid-latitude glaciation on Mars: A proposed climate scenario". Icarus. 203: 300–405.
{{cite journal}}
: Explicit use of et al. in:|first1=
(help) - Mischna, M. et al. 2003. On the orbital forcing of martian water and CO2 cycles: A general circulation model study with simplified volatile schemes. J. Geophys. Res. 108. (E6). 5062.
- Martian gullies could be scientific gold mines. Leonard David, 11/13/2006.
- Head, JW; Marchant, DR; Kreslavsky, MA (2008). "Formation of gullies on Mars: Link to recent climate history and insolation microenvironments implicate surface water flow origin". PNAS. 105 (36): 13258–63. Bibcode:2008PNAS..10513258H. doi:10.1073/pnas.0803760105. PMC 2734344. PMID 18725636.
- "NASA Finds Possible Signs of Flowing Water on Mars". voanews.com. Retrieved August 5, 2011.
- "Is Mars Weeping Salty Tears?". news.sciencemag.org. Retrieved August 5, 2011.
- "Mars Gullies May Have Been Formed By Flowing Liquid Brine". Sciencedaily.com. February 15, 2009. Retrieved February 10, 2013.
- ^ Osterloo, MM; Hamilton, VE; Bandfield, JL; Glotch, TD; Baldridge, AM; Christensen, PR; Tornabene, LL; Anderson, FS (2008). "Chloride-Bearing Materials in the Southern Highlands of Mars". Science. 319 (5870): 1651–1654. Bibcode:2008Sci...319.1651O. doi:10.1126/science.1150690. PMID 18356522.
- ^ Brown, Dwayne; Cole, Steve; Webster, Guy; Agle, D.C. (September 27, 2012). "NASA Rover Finds Old Streambed On Martian Surface". NASA. Retrieved September 28, 2012.
- ^ NASA (September 27, 2012). "NASA's Curiosity Rover Finds Old Streambed on Mars - video (51:40)". NASAtelevision. Retrieved September 28, 2012.
- ^ Chang, Alicia (September 27, 2012). "Mars rover Curiosity finds signs of ancient stream". Associated Press. Retrieved September 27, 2012.
- ^ Morton, Oliver (2002). Mapping Mars: Science, Imagination, and the Birth of a World. New York: Picador USA. p. 98. ISBN 0-312-24551-3. Cite error: The named reference "mapping mars" was defined multiple times with different content (see the help page).
- Online Atlas of Mars
- Catalog Page for PIA03467
- "Online Atlas of Mars". Ralphaeschliman.com. Retrieved December 16, 2012.
- "PIA03467: The MGS MOC Wide Angle Map of Mars". Photojournal. NASA / Jet Propulsion Laboratory. February 16, 2002. Retrieved December 16, 2012.
- "Mars Exploration: Missions". Marsprogram.jpl.nasa.gov. Retrieved December 19, 2010.
- "ch5". History.nasa.gov. Retrieved December 19, 2010.
- "ch7". History.nasa.gov. Retrieved December 19, 2010.
- ^ Raeburn, P. 1998. Uncovering the Secrets of the Red Planet Mars. National Geographic Society. Washington D.C.
- ^ Moore, P. et al. 1990. The Atlas of the Solar System. Mitchell Beazley Publishers NY, NY.
- ^ Arvidson, R; Gooding, James L.; Moore, Henry J. (1989). "The Martian surface as Imaged, Sampled, and Analyzed by the Viking Landers". Review of Geophysics. 27: 39–60. Bibcode:1989RvGeo..27...39A. doi:10.1029/RG027i001p00039.
- Clark, B.; Baird, AK; Rose Jr, HJ; Toulmin P, 3rd; Keil, K; Castro, AJ; Kelliher, WC; Rowe, CD; Evans, PH (1976). "Inorganic Analysis of Martian Samples at the Viking Landing Sites". Science. 194 (4271): 1283–1288. Bibcode:1976Sci...194.1283C. doi:10.1126/science.194.4271.1283. PMID 17797084.
{{cite journal}}
: CS1 maint: numeric names: authors list (link) - Baird, A.; Toulmin P, 3rd; Clark, BC; Rose Jr, HJ; Keil, K; Christian, RP; Gooding, JL (1976). "Mineralogic and Petrologic Implications of Viking Geochemical Results From Mars: Interim Report". Science. 194 (4271): 1288–1293. Bibcode:1976Sci...194.1288B. doi:10.1126/science.194.4271.1288. PMID 17797085.
{{cite journal}}
: CS1 maint: numeric names: authors list (link) - Hoefen, T.; Clark, RN; Bandfield, JL; Smith, MD; Pearl, JC; Christensen, PR (2003). "Discovery of Olivine in the Nili Fossae Region of Mars". Science. 302 (5645): 627–630. Bibcode:2003Sci...302..627H. doi:10.1126/science.1089647. PMID 14576430.
- Hamiliton, W.; Christensen, Philip R.; McSween, Harry Y. (1997). "Determination of Martian meteorite lithologies and mineralogies using vibrational spectroscopy". Journal of Geophysical Research. 102: 25593–25603. Bibcode:1997JGR...10225593H. doi:10.1029/97JE01874.
- Henderson, Mark (December 7, 2006). "Water has been flowing on Mars within past five years, Nasa says". The Times. UK. Retrieved March 17, 2007.
- Mars photo evidence shows recently running water. The Christian Science Monitor. Retrieved on March 17, 2007
- ^ Malin, Michael C.; Edgett, Kenneth S. (2001). "Mars Global Surveyor Mars Orbiter Camera: Interplanetary cruise through primary mission". Journal of Geophysical Research. 106: 23429–23570. Bibcode:2001JGR...10623429M. doi:10.1029/2000JE001455.
- "Mars Global Surveyor MOC2-1618 Release". Msss.com. doi:10.1126/science.288.5475.2330. Retrieved December 19, 2010.
- Malin, M.; Edgett, KS; Posiolova, LV; McColley, SM; Dobrea, EZ (2006). "Present-Day Impact Cratering Rate and Contemporary Gully Activity on Mars". Science. 314 (5805): 1573–1577. Bibcode:2006Sci...314.1573M. doi:10.1126/science.1135156. PMID 17158321.
- "Changing Mars Gullies Hint at Recent Flowing Water". SPACE.com. December 6, 2006. Retrieved December 19, 2010.
- "Mars Global Surveyor MOC2-239 Release". Mars.jpl.nasa.gov. Retrieved December 19, 2010.
- "HiRISE | Slope Streaks in Marte Vallis (PSP_003570_1915)". Hirise.lpl.arizona.edu. Retrieved December 19, 2010.
- "spcae.com". spcae.com. Retrieved December 19, 2010.
- Malin (2010). "An overview of the 1985–2006 Mars Orbiter Camera science investigation". The Mars Journal. 5: 1. Bibcode:2010IJMSE...5....1M. doi:10.1555/mars.2010.0001.
- Zimbelman, J.; Griffin, L. (2010). "HiRISE images of yardangs and sinuous ridges in the lower member of the Medusae Fossae Formation, Mars". Icarus. 205: 198–210. Bibcode:2010Icar..205..198Z. doi:10.1016/j.icarus.2009.04.003.
{{cite journal}}
: Cite has empty unknown parameter:|author-name-separator=
(help); Unknown parameter|author-separator=
ignored (help) - ^ Newsom, H.; Lanza, Nina L.; Ollila, Ann M.; Wiseman, Sandra M.; Roush, Ted L.; Marzo, Giuseppe A.; Tornabene, Livio L.; Okubo, Chris H.; Osterloo, Mikki M. (2010). "Inverted channel deposits on the floor of Miyamoto crater, Mars". Icarus. 205: 64–72. Bibcode:2010Icar..205...64N. doi:10.1016/j.icarus.2009.03.030. Cite error: The named reference "ReferenceB" was defined multiple times with different content (see the help page).
- ^ Weitz, C.; Milliken, R.E.; Grant, J.A.; McEwen, A.S.; Williams, R.M.E.; Bishop, J.L.; Thomson, B.J. (2010). "Mars Reconnaissance Orbiter observations of light-toned layered deposits and associated fluvial landforms on the plateaus adjacent to Valles Marineris". Icarus. 205: 73–102. Bibcode:2010Icar..205...73W. doi:10.1016/j.icarus.2009.04.017.
- ^ "Icarus, Volume 210, Issue 2, Pages 539–1000 (December 2010)". ScienceDirect. Retrieved December 19, 2010.
- ^ Fairen, A.; Davila, AF; Gago-Duport, L; Amils, R; McKay, CP (2009). "Stability against freezing of aqueous solutions on early Mars". Nature. 459 (7245): 401–404. Bibcode:2009Natur.459..401F. doi:10.1038/nature07978. PMID 19458717.
- Atmospheric and Meteorological Properties, NASA
- ^ Golombek, M.; Cook, RA; Economou, T; Folkner, WM; Haldemann, AF; Kallemeyn, PH; Knudsen, JM; Manning, RM; Moore, HJ (1997). "Overview of the Mars Pathfinder Mission and Assessment of Landing Site Predictions". Science. 278 (5344): 1743–1748. Bibcode:1997Sci...278.1743G. doi:10.1126/science.278.5344.1743. PMID 9388167.
- Murche, S.; et al. (1993). "Spatial Variations in the Spectral Properties of Bright Regions on Mars". Icarus. 105 (2): 454–468. Bibcode:1993Icar..105..454M. doi:10.1006/icar.1993.1141.
{{cite journal}}
: Explicit use of et al. in:|first1=
(help) - "Home Page for Bell (1996) Geochemical Society paper". Marswatch.tn.cornell.edu. Retrieved December 19, 2010.
- Feldman, WC; Boynton, WV; Tokar, RL; Prettyman, TH; Gasnault, O; Squyres, SW; Elphic, RC; Lawrence, DJ; Lawson, SL (2002). "Global Distribution of Neutrons from Mars: Results from Mars Odyssey". Science. 297 (5578): 75–78. Bibcode:2002Sci...297...75F. doi:10.1126/science.1073541. PMID 12040088.
- Mitrofanov, I.; Anfimov, D; Kozyrev, A; Litvak, M; Sanin, A; Tret'yakov, V; Krylov, A; Shvetsov, V; Boynton, W (2002). "Maps of Subsurface Hydrogen from the High Energy Neutron Detector, Mars Odyssey". Science. 297 (5578): 78–81. Bibcode:2002Sci...297...78M. doi:10.1126/science.1073616. PMID 12040089.
- Boynton, W.; Feldman, WC; Squyres, SW; Prettyman, TH; Bruckner, J; Evans, LG; Reedy, RC; Starr, R; Arnold, JR (2002). "Distribution of Hydrogen in the Near Surface of Mars: Evidence for Subsurface Ice Deposits". Science. 297 (5578): 81–85. Bibcode:2002Sci...297...81B. doi:10.1126/science.1073722. PMID 12040090.
- ^ Arvidson, P. H.; Tamppari, L.; Arvidson, R. E.; Bass, D.; Blaney, D.; Boynton, W.; Carswell, A.; Catling, D.; Clark, B. (2008). "Introduction to special section on the phoenix mission: Landing site characterization experiments, mission overviews, and expected science". J. Geophysical Research. 113. Bibcode:2008JGRE..11300A18S. doi:10.1029/2008JE003083.
- "The Dirt on Mars Lander Soil Findings". SPACE.com. Retrieved December 19, 2010.
- ^ Head, J.; Neukum, G.; Jaumann, R.; Hiesinger, H.; Hauber, E.; Carr, M.; Masson, P.; Foing, B.; Hoffmann, H. (2005). "Tropical to mid-latitude snow and ice accumulation, flow and glaciation on Mars". Nature. 434 (7031): 346–350. Bibcode:2005Natur.434..346H. doi:10.1038/nature03359. PMID 15772652. Cite error: The named reference "Head, J. 2005" was defined multiple times with different content (see the help page).
- ^ "Mars' climate in flux: Mid-latitude glaciers | Mars Today – Your Daily Source of Mars News". Mars Today. October 17, 2005. Retrieved December 19, 2010. Cite error: The named reference "marstoday.com" was defined multiple times with different content (see the help page).
- ^ Richard Lewis (April 23, 2008). "Glaciers Reveal Martian Climate Has Been Recently Active | Brown University Media Relations". News.brown.edu. Retrieved December 19, 2010. Cite error: The named reference "news.brown.edu" was defined multiple times with different content (see the help page).
- ^ Plaut, Jeffrey J.; Safaeinili, Ali; Holt, John W.; Phillips, Roger J.; Head, James W.; Seu, Roberto; Putzig, Nathaniel E.; Frigeri, Alessandro (2009). "Radar Evidence for Ice in Lobate Debris Aprons in the Mid-Northern Latitudes of Mars" (PDF). Geophysical Research Letters. 36 (2). Bibcode:2009GeoRL..3602203P. doi:10.1029/2008GL036379. Cite error: The named reference "Plaut, J. 2008" was defined multiple times with different content (see the help page).
- ^ Holt, J. W.; Safaeinili, A.; Plaut, J. J.; Young, D. A.; Head, J. W.; Phillips, R. J.; Campbell, B. A.; Carter, L. M.; Gim, Y. (2008). "Radar Sounding Evidence for Ice within Lobate Debris Aprons near Hellas Basin, Mid-Southern Latitudes of Mars" (PDF). Lunar and Planetary Science. XXXIX: 2441. Bibcode:2008LPI....39.2441H. Cite error: The named reference "Holt, J. 2008" was defined multiple times with different content (see the help page).
- ^ Plaut, Jeffrey J.; Safaeinili, Ali; Holt, John W.; Phillips, Roger J.; Head, James W.; Seu, Roberto; Putzig, Nathaniel E.; Frigeri, Alessandro (2009). "Radar evidence for ice in lobate debris aprons in the mid-northern latitudes of Mars" (PDF). Geophysical Research Letters. 36 (2). Bibcode:2009GeoRL..3602203P. doi:10.1029/2008GL036379. Cite error: The named reference "planetary.brown.edu" was defined multiple times with different content (see the help page).
- "Reull Vallis (Released 22 October 2002) | Mars Odyssey Mission THEMIS". Themis.asu.edu. Retrieved December 19, 2010.
- "Dao Vallis (Released 7 August 2002) | Mars Odyssey Mission THEMIS". Themis.asu.edu. Retrieved December 19, 2010.
- Mellon, M.; Jakosky, B. (1993). "Geographic variations in the thermal and diffusive stability of ground ice on Mars". J. Geographical Research. 98: 3345–3364. Bibcode:1993JGR....98.3345M. doi:10.1029/92JE02355.
{{cite journal}}
: Cite has empty unknown parameter:|author-name-separator=
(help); Unknown parameter|author-separator=
ignored (help) - Johnson, John (August 1, 2008). "There's water on Mars, NASA confirms". Los Angeles Times. Retrieved August 1, 2008.
- Heldmann, Jennifer L.; et al. (May 7, 2005). "Formation of Martian gullies by the action of liquid water flowing under current Martian environmental conditions" (PDF). Journal of Geophysical Research. 110: Eo5004. Bibcode:2005JGRE..11005004H. doi:10.1029/2004JE002261. Retrieved September 14, 2008Template:Inconsistent citations
{{cite journal}}
: Explicit use of et al. in:|last=
(help)CS1 maint: postscript (link) 'conditions such as now occur on Mars, outside of the temperature-pressure stability regime of liquid water' ... 'Liquid water is typically stable at the lowest elevations and at low latitudes on the planet because the atmospheric pressure is greater than the vapor pressure of water and surface temperatures in equatorial regions can reach 273 K for parts of the day ' - Kostama, V.-P.; Kreslavsky, M. A.; Head, J. W. (June 3, 2006). "Recent high-latitude icy mantle in the northern plains of Mars: Characteristics and ages of emplacement". Geophysical Research Letters. 33 (11): L11201. Bibcode:2006GeoRL..3311201K. doi:10.1029/2006GL025946. Retrieved August 12, 2007Template:Inconsistent citations
{{cite journal}}
: CS1 maint: postscript (link) 'Martian high-latitude zones are covered with a smooth, layered ice-rich mantle' - Hecht, MH; Kounaves, SP; Quinn, RC; West, SJ; Young, SM; Ming, DW; Catling, DC; Clark, BC; Boynton, WV (2009). "Detection of Perchlorate and the Soluble Chemistry of Martian Soil at the Phoenix Lander Site". Science. 325 (5936): 64–67. Bibcode:2009Sci...325...64H. doi:10.1126/science.1172466. PMID 19574385.
- ^ Chang, Kenneth (2009) Blobs in Photos of Mars Lander Stir a Debate: Are They Water?, New York Times (online), March 16, 2009, retrieved April 4, 2009;
- http://articles.latimes.com/2009/mar/14/nation/na-marswater12.
{{cite news}}
: Missing or empty|title=
(help) - "Astrobiology Top 10: Too Salty to Freeze". Astrobio.net. Retrieved December 19, 2010.
- Smith, PH; Tamppari, LK; Arvidson, RE; Bass, D; Blaney, D; Boynton, WV; Carswell, A; Catling, DC; Clark, BC (2009). "H2O at the Phoenix Landing Site". Science. 325 (5936): 58–61. Bibcode:2009Sci...325...58S. doi:10.1126/science.1172339. PMID 19574383.
- "The Dirt on Mars Lander Soil Findings". Space.com. Retrieved December 19, 2010.
- "CSA – News Release". Asc-csa.gc.ca. July 2, 2009. Retrieved December 19, 2010.
- Boynton, WV; Ming, DW; Kounaves, SP; Young, SM; Arvidson, RE; Hecht, MH; Hoffman, J; Niles, PB; Hamara, DK (2009). "Evidence for Calcium Carbonate at the Mars Phoenix Landing Site". Science. 325 (5936): 61–64. Bibcode:2009Sci...325...61B. doi:10.1126/science.1172768. PMID 19574384.
- "Audio Recording of Phoenix Media Telecon for Aug. 5, 2008". Jet Propulsion Laboratory. NASA. August 5, 2008. Retrieved July 14, 2009.
- NASA Data Shed New Light About Water and Volcanoes on Mars
- "Mars Exploration Rover Mission: Press Releases". Marsrovers.jpl.nasa.gov. March 5, 2004. Retrieved December 19, 2010.
- "NASA - Mars Rover Spirit Unearths Surprise Evidence of Wetter Past". Nasa.gov. May 21, 2007. Retrieved February 10, 2013.
- Amos, Jonathan (December 11, 2007). "Mars robot unearths microbe clue". NASA says its robot rover Spirit has made one of its most significant discoveries on the surface of Mars. BBC News. Retrieved December 12, 2007.
- Bertster, Guy (December 10, 2007). "Mars Rover Investigates Signs of Steamy Martian Past". Press Release. Jet Propulsion Laboratory, Pasadena, California. Retrieved December 12, 2007.
- ^ "Opportunity Rover Finds Strong Evidence Meridiani Planum Was Wet". Retrieved July 8, 2006.
- Klingelhofer, G., et al. (2005) Lunar Planet. Sci. XXXVI abstr. 2349
- Schroder, C., et al. (2005) European Geosciences Union, General Assembly, Geophysical Research abstr., Vol. 7, 10254, 2005
- Morris,S., et al. Mossbauer mineralogy of rock, soil, and dust at Gusev crater, Mars: Spirit’s journal through weakly altered olivine basalt on the plains and pervasively altered basalt in the Columbia Hills. J. Geophys. Res: 111
- Ming,D., et al. 2006 Geochemical and mineralogical indicators for aqueous processes in the Columbia Hills of Gusev crater, Mars. J. Geophys. Res.111
- Bell, J (ed.) The Martian Surface. 2008. Cambridge University Press. ISBN 978-0-521-86698-9
- "Outcrop of long-sought rare rock on Mars found". Sciencedaily.com. June 4, 2010. doi:10.1126/science.1189667. Retrieved February 10, 2013.
- Richard V. Morris, Steven W. Ruff, Ralf Gellert, Douglas W. Ming, Raymond E. Arvidson, Benton C. Clark, D. C. Golden, Kirsten Siebach, Göstar Klingelhöfer, Christian Schröder, Iris Fleischer, Albert S. Yen, Steven W. Squyres. Identification of Carbonate-Rich Outcrops on Mars by the Spirit Rover. Science, June 3, 2010 doi:10.1126/science.1189667
- Brown, Dwayne; Webster, Guy; Jones, Nance Neal (December 3, 3012). "NASA Mars Rover Fully Analyzes First Martian Soil Samples". NASA. Retrieved December 3, 2012.
{{cite web}}
: Check date values in:|date=
(help) - Chang, Ken (December 3, 2012). "Mars Rover Discovery Revealed". New York Times. Retrieved December 3, 2012.
- ^ Webster, Guy; Brown, Dwayne (March 18, 2013). "Curiosity Mars Rover Sees Trend In Water Presence". NASA. Retrieved March 20, 2013.
- Rincon, Paul (March 19, 2013). "Curiosity breaks rock to reveal dazzling white interior". BBC. Retrieved March 19, 2013.
- Staff (March 20, 2013). "Red planet coughs up a white rock, and scientists freak out". MSN. Retrieved March 20, 2013.
- "HiRISE | Sinuous Ridges Near Aeolis Mensae". Hiroc.lpl.arizona.edu. January 31, 2007. Retrieved December 19, 2010.
- "HiRISE | High Resolution Imaging Science Experiment". Hirise.lpl.arizona.edu?psp_008437_1750. Retrieved December 19, 2010.
- Grotzinger, J. and R. Milliken (eds.) 2012. Sedimentary Geology of Mars. SEPM
- "Target Zone: Nilosyrtis? | Mars Odyssey Mission THEMIS". Themis.asu.edu. Retrieved December 19, 2010.
- ^ Head, James W.; Mustard, John F.; Kreslavsky, Mikhail A.; Milliken, Ralph E.; Marchant, David R. (2003). "Recent ice ages on Mars". Nature. 426 (6968): 797–802. doi:10.1038/nature02114. PMID 14685228.
- Head, J. et al. 2008. Formation of gullies on Mars: Link to recent climate history and insolation microenvironments implicate surface water flow origin. PNAS: 105. 13258-13263.
- ^ MLA NASA/Jet Propulsion Laboratory (2003, December 18). Mars May Be Emerging From An Ice Age. ScienceDaily. Retrieved February 19, 2009, from http://www.sciencedaily.com /releases/2003/12/031218075443.htmAds by GoogleAdvertise Cite error: The named reference "sciencedaily.com" was defined multiple times with different content (see the help page).
- Malin, M.; Edgett, KS; Posiolova, LV; McColley, SM; Dobrea, EZ (2006). "Present-day impact cratering rate and contemporary gully activity on Mars". Science. 314 (5805): 1573–1577. Bibcode:2006Sci...314.1573M. doi:10.1126/science.1135156. PMID 17158321.
- Kolb, K.; Pelletier, Jon D.; McEwen, Alfred S. (2010). "Modeling the formation of bright slope deposits associated with gullies in Hale Crater, Mars: Implications for recent liquid water". Icarus. 205: 113–137. Bibcode:2010Icar..205..113K. doi:10.1016/j.icarus.2009.09.009.
- Byrne, S; Dundas, CM; Kennedy, MR; Mellon, MT; McEwen, AS; Cull, SC; Daubar, IJ; Shean, DE; Seelos, KD (2009). "Distribution of mid-latitude ground ice on Mars from new impact craters". Science. 325 (5948): 1674–1676. Bibcode:2009Sci...325.1674B. doi:10.1126/science.1175307. PMID 19779195.
- "Water Ice Exposed in Mars Craters". SPACE.com. Retrieved December 19, 2010.
- http://nasa.gov/mission/MRO/news/mro20090924.html
- Milazzo, M.; Keszthelyi, L.P.; Jaeger, W.L.; Rosiek, M.; Mattson, S.; Verba, C.; Beyer, R.A.; Geissler, P.E.; McEwen, A.S. (2009). "The discovery of columnar jointing on Mars". Geology. 37 (2): 171–174. doi:10.1130/G25187A.1.
- Milazzo, M.; Keszthelyi, L. P.; McEwen, A. S.; Jaeger, W. (2003). "The formation of columnar joints on Earth and Mars (abstract #2120)" (PDF). Lunar and Planetary Science. XXXIV: 2120. Bibcode:2003LPI....34.2120M.
- Mangold, C.; Quantin, C; Ansan, V; Delacourt, C; Allemand, P (2004). "Evidence for precipitation on Mars from dendritic valleys in the Valles Marineris area". Science. 305 (5680): 78–81. Bibcode:2004Sci...305...78M. doi:10.1126/science.1097549. PMID 15232103.
- Murchie, Scott; Roach, Leah; Seelos, Frank; Milliken, Ralph; Mustard, John; Arvidson, Raymond; Wiseman, Sandra; Lichtenberg, Kimberly; Andrews-Hanna, Jeffrey (2009). "Evidence for the origin of layered deposits in Candor Chasma, Mars, from mineral composition and hydrologic modeling". Journal of Geophysical Research. 114. Bibcode:2009JGRE..11400D05M. doi:10.1029/2009JE003343.
- Edgett, E. (2005). "The sedimentary rocks of Sinus Meridiani: Five key observations from data acquired by the Mars Global Surveyor and Mars Odyssey orbiters". Mars. 1: 5–58. Bibcode:2005Mars....1....5E. doi:10.1555/mars.2005.0002.
- Hartmann, W. 2003. A Traveler's Guide to Mars. Workman Publishing. NY NY.
- Andrews‐Hanna, J. C., R. J. Phillips, and M. T. Zuber (2007), Meridiani Planum and the global hydrology of Mars, Nature, 446, 163–166, doi:10.1038/nature05594.
- Andrews‐Hanna, J. C., M. T. Zuber, R. E. Arvidson, and S. M. Wiseman (2010), Early Mars hydrology: Meridiani playa deposits and the sedimentary record of Arabia Terra, J. Geophys. Res., 115, E06002, doi:10.1029/2009JE003485.
- Grotzinger, J. P., et al. (2005), Stratigraphy and sedimentology of a dry to wet eolian depositional system, Burns formation, Meridiani Planum, Mars, Earth Planet. Sci. Lett., 240, 11–72, doi:10.1016/j.epsl.2005.09.039
- McLennan, S. M., et al. (2005), Provenance and diagenesis of the evaporitebearing Burns formation, Meridiani Planum, Mars, Earth Planet. Sci. Lett., 240, 95–121, doi:10.1016/j.epsl.2005.09.041
- Squyres, S. W., and A. H. Knoll (2005), Sedimentary rocks at Meridiani Planum: Origin, diagenesis, and implications for life on Mars, Earth Planet. Sci. Lett., 240, 1–10, doi:10.1016/j.epsl.2005.09.038.
- Squyres, S. W., et al. (2006), Two years at Meridiani Planum: Results from the Opportunity rover, Science, 313, 1403–1407, doi:10.1126/science.
- M. Wiseman, J. C. Andrews-Hanna, R. E. Arvidson3, J. F. Mustard, K. J. Zabrusky DISTRIBUTION OF HYDRATED SULFATES ACROSS ARABIA TERRA USING CRISM DATA: IMPLICATIONS FOR MARTIAN HYDROLOGY. 42nd Lunar and Planetary Science Conference (2011) 2133.pdf
- ^ "Water ice in crater at Martian north pole" – July 27, 2005 ESA Press release. Retrieved March 17, 2006. Cite error: The named reference "lake" was defined multiple times with different content (see the help page).
- ^ "Ice lake found on the Red Planet" – July 29, 2005 BBC story. Retrieved March 17, 2006. Cite error: The named reference "BBClake" was defined multiple times with different content (see the help page).
- ^ Cabrol, N. and E. Grin (eds.). 2010. Lakes on Mars. Elsevier. NY
- ^ Murray, John B. (2005). "Evidence from the Mars Express High Resolution Stereo Camera for a frozen sea close to Mars' equator". Nature. 434 (7031): 352–356. Bibcode:2005Natur.434..352M. doi:10.1038/nature03379. PMID 15772653.
{{cite journal}}
: Unknown parameter|coauthors=
ignored (|author=
suggested) (help) Cite error: The named reference "Murray2007" was defined multiple times with different content (see the help page). - Orosei, R.; Cartacci, M.; Cicchetti, A.; Noschese, R.; Federico, C.; Frigeri, A.; Flamini, E.; Holt, J. W.; Marinangeli, L. (2008). "Radar subsurface sounding over the putative frozen sea in Cerberus Palus, Mars" (PDF). Lunar and Planetary Science. XXXIX: 1. Bibcode:2007AGUFM.P14B..05O. doi:10.1109/ICGPR.2010.5550143. ISBN 978-1-4244-4604-9.
- ISBN 978-0-521-85226-5
- "ESA – Mars Express – Breathtaking views of Deuteronilus Mensae on Mars". Esa.int. March 14, 2005. Retrieved December 19, 2010.
- "HiRISE | Glacier? (ESP_018857_2225)". Uahirise.org. Retrieved December 19, 2010.
- Shean, David E. (2005). "Origin and evolution of a cold-based tropical mountain glacier on Mars: The Pavonis Mons fan-shaped deposit". Journal of Geophysical Research. 110. Bibcode:2005JGRE..11005001S. doi:10.1029/2004JE002360.
- "HiRISE | Fretted Terrain Valley Traverse (PSP_009719_2230)". Hirise.lpl.arizona.edu. Retrieved December 19, 2010.
- ^ "Mars' South Pole Ice Deep and Wide". NASA News & Media Resources. NASA. March 15, 2007. Retrieved March 18, 2013.
{{cite web}}
: External link in
(help)|work=
- ^ "Water at Martian south pole". European Space Agency (ESA). March 17, 2004. Retrieved September 11, 2009. Cite error: The named reference "ESAwater" was defined multiple times with different content (see the help page).
- Kostama, V.-P.; Kreslavsky, M. A.; Head, J. W. (June 3, 2006). "Recent high-latitude icy mantle in the northern plains of Mars: Characteristics and ages of emplacement". Geophysical Research Letters. 33 (11): L11201. Bibcode:2006GeoRL..3311201K. doi:10.1029/2006GL025946. Retrieved August 1, 2008Template:Inconsistent citations
{{cite journal}}
: CS1 maint: postscript (link) - "Radar Map of Buried Mars Layers Matches Climate Cycles". OnOrbit. Retrieved December 19, 2010.
- "Polygonal Patterned Ground: Surface Similarities Between Mars and Earth | SpaceRef – Your Space Reference". SpaceRef. September 28, 2002. Retrieved December 19, 2010.
- Squyres, S. (1989). "Urey Prize Lecture: Water on Mars". Icarus. 79 (2): 229–288. Bibcode:1989Icar...79..229S. doi:10.1016/0019-1035(89)90078-X.
- Lefort, A.; Russell, P.S.; Thomas, N. (2010). "Scaloped terrains in the Peneus and Amphitrites Paterae region of Mars as observed by HiRISE". Icarus. 205: 259–268. Bibcode:2010Icar..205..259L. doi:10.1016/j.icarus.2009.06.005.
- "NASA – Turbulent Lava Flow in Mars' Athabasca Valles". Nasa.gov. January 11, 2010. Retrieved December 19, 2010.
- "HiRISE | Dissected Mantled Terrain (PSP_002917_2175)". Hirise.lpl.arizona.edu. Retrieved December 19, 2010.
- Lefort, A.; Russell, P.S.; Thomas, N. (2010). "Scalloped terrains in the Peneus and Amphitrites Paterae region of Mars as observed by HiRISE". Icarus. 205: 259–268. Bibcode:2010Icar..205..259L. doi:10.1016/j.icarus.2009.06.005.
- NASA Spacecraft Data Suggest Water Flowing on Mars, NASA, August 4, 2011
- "Shergotty Meteorite – JPL, NASA". .jpl.nasa.gov. Retrieved December 19, 2010.
- Treiman, A (2005). "The nakhlite meteorites: Augite-rich igneous rocks from Mars" (PDF). Chemie der Erde – Geochemistry. 65 (3): 203. Bibcode:2005ChEG...65..203T. doi:10.1016/j.chemer.2005.01.004. Retrieved September 8, 2006.
- McKay, D.; Gibson Jr, EK; Thomas-Keprta, KL; Vali, H; Romanek, CS; Clemett, SJ; Chillier, XD; Maechling, CR; Zare, RN (1996). "Search for Past Life on Mars: Possible Relic Biogenic Activity in Martian Meteorite AL84001". Science. 273 (5277): 924–930. Bibcode:1996Sci...273..924M. doi:10.1126/science.273.5277.924. PMID 8688069.
- Gibbs, W.; Powell, C. (August 19, 1996). "Bugs in the Data?". Scientific American.
- "Controversy Continues: Mars Meteorite Clings to Life – Or Does It?". SPACE.com. March 20, 2002. Retrieved December 19, 2010.
- Bada, J.; Glavin, DP; McDonald, GD; Becker, L (1998). "A Search for Endogenous Amino Acids in Martian Meteorite AL84001". Science. 279 (5349): 362–365. Bibcode:1998Sci...279..362B. doi:10.1126/science.279.5349.362. PMID 9430583.
- Goldspiel, J.; Squires, S. (2000). "Groundwater sapping and valley formation on Mars". Icarus. 148: 176–192. Bibcode:2000Icar..148..176G. doi:10.1006/icar.2000.6465.
{{cite journal}}
: Cite has empty unknown parameter:|author-name-separator=
(help); Unknown parameter|author-separator=
ignored (help) - McCauley, J. 1978. Geologic map of the Coprates quadrangle of Mars. U.S. Geol. Misc. Inv. Map I-897
- Nedell, S.; Squyres, Steven W.; Andersen, David W. (1987). "Origin and evolution of the layered deposits in the Valles Marineris, Mars". Icarus. 70 (3): 409–441. Bibcode:1987Icar...70..409N. doi:10.1016/0019-1035(87)90086-8.
- "Spectacular Mars images reveal evidence of ancient lakes". Sciencedaily.com. January 4, 2010. Retrieved December 19, 2010.
- Gupta, Sanjeev; Warner, Nicholas; Kim, Rack; Lin, Yuan; Muller, Jan; -1#Jung-, Shih- (2010). "Hesperian equatorial thermokarst lakes in Ares Vallis as evidence for transient warm conditions on Mars". Geology. 38: 71–74. doi:10.1130/G30579.1.
{{cite journal}}
: CS1 maint: numeric names: authors list (link) - ^ Di Achille, Gaetano; Hynek, Brian M. (2010). "Ancient ocean on Mars supported by global distribution of deltas and valleys". Nature Geoscience. 3 (7): 459. Bibcode:2010NatGe...3..459D. doi:10.1038/ngeo891. Cite error: The named reference "ReferenceA" was defined multiple times with different content (see the help page).
- Clifford, S. M.; Parker, T. J. (2001). "The Evolution of the Martian Hydrosphere: Implications for the Fate of a Primordial Ocean and the Current State of the Northern Plains". Icarus. 154: 40–79. Bibcode:2001Icar..154...40C. doi:10.1006/icar.2001.6671.
{{cite journal}}
: Cite has empty unknown parameter:|author-name-separator=
(help); Unknown parameter|author-separator=
ignored (help) - ^ Baker, V. R.; Strom, R. G.; Gulick, V. C.; Kargel, J. S.; Komatsu, G.; Kale, V. S. (1991). "Ancient oceans, ice sheets and the hydrological cycle on Mars". Nature. 352 (6336): 589–594. Bibcode:1991Natur.352..589B. doi:10.1038/352589a0.
{{cite journal}}
: Cite has empty unknown parameter:|author-name-separator=
(help); Unknown parameter|author-separator=
ignored (help) Cite error: The named reference "Baker" was defined multiple times with different content (see the help page). - ^ Read, Peter L.; Lewis, S. R. (2004). The Martian Climate Revisited: Atmosphere and Environment of a Desert Planet (Paperback). Chichester, UK: Praxis. ISBN 978-3-540-40743-0. Retrieved December 19, 2010.
- "Martian North Once Covered by Ocean". Astrobio.net. Retrieved December 19, 2010.
- "New Map Bolsters Case for Ancient Ocean on Mars". SPACE.com. November 23, 2009. Retrieved December 19, 2010.
- Zuber, Maria T. (2007). "Planetary Science: Mars at the tipping point". Nature. 447 (7146): 785–786. Bibcode:2007Natur.447..785Z. doi:10.1038/447785a. PMID 17568733.
- Smith, D.; et al. (1999). "The Gravity Field of Mars: Results from Mars Global Surveyor" (PDF). Science. 284 (5437): 94–97. Bibcode:1999Sci...286...94S. doi:10.1126/science.286.5437.94.
{{cite journal}}
: Explicit use of et al. in:|first1=
(help) - "Ancient ocean may have covered third of Mars". Sciencedaily.com. June 14, 2010. Retrieved December 19, 2010.
- Fairén, Alberto G.; Davila, Alfonso F.; Gago-Duport, Luis; Haqq-Misra, Jacob D.; Gil, Carolina; McKay, Christopher P.; Kasting, James F. (August 28, 2011). "Cold glacial oceans would have inhibited phyllosilicate sedimentation on early Mars". Nature Geoscience. 4 (10): 667. Bibcode:2011NatGe...4..667F. doi:10.1038/ngeo1243.
- "Mars Ocean Hypothesis Hits the Shore " Articles " NASA Astrobiology". Astrobiology.nasa.gov. January 26, 2001. Retrieved December 19, 2010.
- Kerr, Richard A. (2007). "Is Mars Looking Drier and Drier for Longer and Longer?". Science. 317 (5845): 1673. doi:10.1126/science.317.5845.1673. PMID 17885108.
- Cabrol, N.; Grin, E. (2001). "The Evolution of Lacustrine Environments on Mars: Is Mars Only Hydrologically Dormant?". Icarus. 149 (2): 291–328. Bibcode:2001Icar..149..291C. doi:10.1006/icar.2000.6530.
{{cite journal}}
: Cite has empty unknown parameter:|author-name-separator=
(help); Unknown parameter|author-separator=
ignored (help) - "Once-Habitable Lake Found on Mars". SPACE.com. March 6, 2008. Retrieved December 19, 2010.
- Gulick, V.; Baker, V. (1989). "Fluvial valleys and martian palaeoclimates". Nature. 341 (6242): 514–516. Bibcode:1989Natur.341..514G. doi:10.1038/341514a0.
{{cite journal}}
: Cite has empty unknown parameter:|author-name-separator=
(help); Unknown parameter|author-separator=
ignored (help) - Head, J.; Kreslavsky, M. A.; Ivanov, M. A.; Hiesinger, H.; Fuller, E. R.; Pratt, S. (2001). "Water in Middle Mars History: New Insights From MOLO Data". American Geophysical Union. Bibcode:2001AGUSM...P31A02H.
- Head, J.; et al. (2001). "Exploration for standing Bodies of Water on Mars: When Were They There, Where did They go, and What are the Implications for Astrobiology?". American Geophysical Union. 21: 03. Bibcode:2001AGUFM.P21C..03H.
{{cite journal}}
: Explicit use of et al. in:|first1=
(help) - "Mars Rover's Meteorite Discovery Triggers Questions". Space.com. Retrieved February 10, 2013.
- ^ Source: NASA HQ Posted Tuesday, October 28, 2008 (October 28, 2008). "NASA Mars Reconnaissance Orbiter Reveals Details of a Wetter Mars | SpaceRef - Your Space Reference". SpaceRef. Retrieved February 10, 2013.
{{cite web}}
: CS1 maint: multiple names: authors list (link) CS1 maint: numeric names: authors list (link) - "Amazing Mars: Discoveries in 2008". Space.com. December 30, 2008. Retrieved February 10, 2013.
- "What Mars Fossils Might Look Like". SPACE.com. May 1, 2008. Retrieved December 19, 2010.
- http://blogs.discover magazine.com/80beats/2008/05/30/mars-water-suited-for-pickles-not-for-life-2/
- Mittlefehldt, D. (1994). "ALH84001, a cumulate orthopyroxenite member of the martian meteorite clan". Meteortics. 29: 214–221.
- Boston, P.; Ivanov, MV; McKay, CP (1992). "On the Possibility of Chemosynthetic Ecosystems in Subsurface Habitats on Mars". Icarus. 95 (2): 300–308. Bibcode:1992Icar...95..300B. doi:10.1016/0019-1035(92)90045-9. PMID 11539823.
- Thompson, Andrea (April 14, 2009). "Mars Sprinkled with Salty Mysteries". SPACE.com. Retrieved October 9, 2012.
- Jpl.Nasa.Gov (July 2, 2009). "NASA Phoenix Results Point to Martian Climate Cycles - NASA Jet Propulsion Laboratory". Jpl.nasa.gov. Retrieved February 10, 2013.
- . A. C. Schuerger, D. W. Ming, and D. C. Golden LOW BIOTOXICITIES OF ANALOG SOILS SUGGEST THAT THE SURFACE OF MARS MAY BE HABITABLE FOR TERRESTRIAL MICROORGANISMS 43rd Lunar and Planetary Science Conference (2012)
- "Astrobiology Magazine". Astrobio.net. Retrieved December 19, 2010.
- Cowen, R. (2003). "Martian Invasion". Science News. 164 (19): 298–300. doi:10.2307/4018828. JSTOR 4018828.
- McKay, C. P. (1997). "Looking for life on Mars". Astronomy. 25 (8): 38–43. Bibcode:1997Ast....25...38F.
- Gilichinsky, D.; Wilson, GS; Friedmann, EI; McKay, CP; Sletten, RS; Rivkina, EM; Vishnivetskaya, TA; Erokhina, LG; Ivanushkina, NE (2007). "Microbal Populations in Antarctic Permafrost: Biodiversity, State, Age, and Implication for Astrobiology". Astrobiology. 7 (2): 275–311. Bibcode:2007AsBio...7..275G. doi:10.1089/ast.2006.0012. PMID 17480161.
- Raeburn, P. 1998. Mars. National Geographic Society. Washington, D.C.
- Allen, C.; Albert, FG; Chafetz, HS; Combie, J; Graham, CR; Kieft, TL; Kivett, SJ; McKay, DS; Steele, A (2000). "Microscopic Physical Biomarkers in Carbonate Hot Springs: Implications in the Search fo Life on Mars". Icarus. 147 (1): 49–67. Bibcode:2000Icar..147...49A. doi:10.1006/icar.2000.6435. PMID 11543582.
- Fredrickson, J.; Onstott, T. (1996). "Microbes Deep inside the Earth". Scientific American. 275 (4): 68–73. doi:10.1038/scientificamerican1096-68. PMID 8797299.
{{cite journal}}
: Cite has empty unknown parameter:|author-name-separator=
(help); Unknown parameter|author-separator=
ignored (help) - Pedersen, K. (1993). "The deep subterranean biosphere". Earth-Science Reviews. 34 (4): 243–260. Bibcode:1993ESRv...34..243P. doi:10.1016/0012-8252(93)90058-F.
- Stevens, T; McKinley, J. (1995). "Lithoautotrophic Microbial Ecosystems in Deep Basalt Aquifers". Science. 270 (5235): 450–454. Bibcode:1995Sci...270..450S. doi:10.1126/science.270.5235.450.
{{cite journal}}
: Cite has empty unknown parameter:|author-name-separator=
(help); Unknown parameter|author-separator=
ignored (help) - Payne, M; Farmer, J. (2001). "Volcanic-Ice Interactions and the Exploration for Extant Martian Life". American Geophysical Union. 22: 0549. Bibcode:2001AGUFM.P22B0549P.
- "Martian Life Appears Less Likely : Discovery News". Dsc.discovery.com. August 12, 2009. Retrieved December 19, 2010.
- "Tough Microbe Has The Right Stuff for Mars". LiveScience. July 18, 2009. Retrieved February 10, 2013.
- Huber, R.; Stotters, P.; Cheminee, J. L.; Richnow, H. H.; Stetter, K. O. (1990). "Hyperthermophilic archaebacteria within the crater and open-sea plume of erupting Macdonald Seamount". Nature. 345 (6271): 179–182. Bibcode:1990Natur.345..179H. doi:10.1038/345179a0.
- Walter, M.; DesMarais, D. (1993). "Preservation of Biological Information in Thermal Spring Deposits: Developing a Strategy for the Search for Fossil Life on Mars". Icarus. 101 (1): 129–143. Bibcode:1993Icar..101..129W. doi:10.1006/icar.1993.1011. PMID 11536937.
{{cite journal}}
: Cite has empty unknown parameter:|author-name-separator=
(help); Unknown parameter|author-separator=
ignored (help) - Allen, C.; Oehler, D. (2008). "A Case for Ancient Springs in Arabia Terra, Mars". Astrobiology. 8 (6): 1093–1112. Bibcode:2008AsBio...8.1093A. doi:10.1089/ast.2008.0239. PMID 19093802.
{{cite journal}}
: Cite has empty unknown parameter:|author-name-separator=
(help); Unknown parameter|author-separator=
ignored (help) - "Evidence of Ancient Hot Springs on Mars Detailed in Astrobiology Journal | SpaceRef – Your Space Reference". SpaceRef. February 11, 2009. Retrieved December 19, 2010.
- Wallace, D.; Sagan, C. (1979). "Evaporation of Ice in Planetary Atmospheres: Ice-Covered Rivers on Mars". Icarus. 39 (3): 385–400. Bibcode:1979Icar...39..385W. doi:10.1016/0019-1035(79)90148-9.
{{cite journal}}
: Cite has empty unknown parameter:|author-name-separator=
(help); Unknown parameter|author-separator=
ignored (help) - Duxbury, N. S.; Zotikov, I. A.; Nealson, K. H.; Romanovsky, V. E.; Carsey, F. D. (2001). "A numerical model for an alternative origin of Lake Vostok and its exobiological implications for Mars" (PDF). Journal of Geophysical Research. 106: 1453. Bibcode:2001JGR...106.1453D. doi:10.1029/2000JE001254. Retrieved April 8, 2009.
- Segura, T. et al. 2001. Effects of Large Impacts on Mars: Implication for River Formation. American Astronomical society, DPS meeting
- Segura, T.; Toon, OB; Colaprete, A; Zahnle, K (2002). "Environmental Effects of Large Impacts on Mars". Science. 298 (5600): 1977–1980. Bibcode:2002Sci...298.1977S. doi:10.1126/science.1073586. PMID 12471254.
- Baker, V.; Milton, D. (1974). "Erosion by Catastrophic Floods on Mars and Earth". Icarus. 23: 27–41. Bibcode:1974Icar...23...27B. doi:10.1016/0019-1035(74)90101-8.
{{cite journal}}
: Cite has empty unknown parameter:|author-name-separator=
(help); Unknown parameter|author-separator=
ignored (help) - Christensen, P. (2005). "The Many Faces of Mars". Scientific American. 293 (1): 32–39. doi:10.1038/scientificamerican0705-32. PMID 16008291.
- Source: Ames Research Center Posted Saturday, June 6, 2009 (June 6, 2009). "NASA Scientists Find Evidence for Liquid Water on a Frozen Early Mars | SpaceRef – Your Space Reference". SpaceRef. Retrieved December 19, 2010.
{{cite web}}
: CS1 maint: multiple names: authors list (link) CS1 maint: numeric names: authors list (link) - Kreslavsky, M.; Head, James W.; Marchant, David R. (2006). "Periods of Active Permafrost Layer Formation During the Geological History of Mars: Implication for Circum-Polar and Mid-Latitude surface Processes" (PDF). Planetary and space Science Special Issue on Polar Processes. 56 (2): 266–288. Bibcode:2008P&SS...56..289K. doi:10.1016/j.pss.2006.02.010.
- "Dead Spacecraft on Mars Lives on in New Study". SPACE.com. June 10, 2008. Retrieved December 19, 2010.
- Lobitz, B.; Wood, BL; Averner, MM; McKay, CP (2001). "Use of spacecraft data to derive regions on Mars where liquid water would be stable". Proc. Natl. Acad. Sci. 98 (5): 2132–2137. Bibcode:2001PNAS...98.2132L. doi:10.1073/pnas.031581098. PMC 30104. PMID 11226204.
- Haberie, Robert M.; McKay, Christopher P.; Schaeffer, James; Cabrol, Nathalie A.; Grin, Edmon A.; Zent, Aaron P.; Quinn, Richard (2001). "On the possibility of liquid water on present-day Mars". J. Geophysical Research. 106: 23317–23326. Bibcode:2001JGR...10623317H. doi:10.1029/2000JE001360.
- Nancy Atkinson (September 4, 2008). "Phoenix Probe Says Both Yes and No to Water on Mars". Universetoday.com. Retrieved December 19, 2010.
- http://www.newscientist.com/article/mg20427373.700 (subscription required)
- Tudor Vieru (December 7, 2009). "Greenhouse Effect on Mars May Be Allowing for Life". News.softpedia.com. Retrieved August 20, 2011.
- Possible New Mars Caves Targets in Search for Life
- Michael T. Mellon Subsurface Ice at Mars: A review of ice and water in the equatorial regions University of Colorado 10 May 2011 Planetary Protection Subcommittee Meeting
- Robert Roy Britt Ice Packs and Methane on Mars Suggest Present Life Possiblespace.com 22 February 2005
- Mellon, M. T., B. M. Jakosky, and S. E. Postawko (1997)The persistence of equatorial ground ice on Mars, J. Geophys. Res., 102(E8), 19357–19369, doi:10.1029/97JE01346.
- John D. Arfstrom A Conceptual Model of Equatorial Ice Sheets on Mars. J Comparative Climatology of Terrestrial Planets (2012)
- Surviving the conditions on Mars DLR, 26 April 2012
- Jean-Pierre de Vera Lichens as survivors in space and on Mars Fungal Ecology Volume 5, Issue 4, August 2012, Pages 472–479
- R. de la Torre Noetzel, F.J. Sanchez Inigo, E. Rabbow, G. Horneck, J. P. de Vera, L.G. Sancho Survival of lichens to simulated Mars conditions
- F.J. Sáncheza, , , E. Mateo-Martíb, J. Raggioc, J. Meeßend, J. Martínez-Fríasb, L.Ga. Sanchoc, S. Ottd, R. de la Torrea The resistance of the lichen Circinaria gyrosa (nom. provis.) towards simulated Mars conditions—a model test for the survival capacity of an eukaryotic extremophile Planetary and Space Science Volume 72, Issue 1, November 2012, Pages 102–110
- ed, Hugh H. Kieffer ... (1994). Mars (. ed.). Tucson : Univ. of Arizona Press. ISBN 0-8165-1257-4.
- Jakosky, Bruce M. (1999). "Water, Climate, and Life". Science. 283 (5402): 648–649. doi:10.1126/science.283.5402.648. PMID 9988657.
{{cite journal}}
: Cite has empty unknown parameters:|month=
and|coauthors=
(help) - "Mars Global Surveyor MOC2-862 Release". Msss.com. Retrieved January 16, 2012.
- "Ancient ocean may have covered third of Mars". Sciencedaily.com. June 13, 2010. Retrieved January 16, 2012.
- Carr, M.H. (1979). "Formation of Martian flood features by relaease of water from confined aquifers". J. Geophys. Res. 84: 2995–3007. Bibcode:1979JGR....84.2995C. doi:10.1029/JB084iB06p02995.
- Craddock, R.A. and Howard, A.D. (2002). The case for rainfall on a warm, wet early Mars. J. Geophys. Res., 107(E11), doi:10.1029/2001JE001505.
- "Flashback: Water on Mars Announced 10 Years Ago". Space.com. Retrieved January 16, 2012.
- Malin, Michael C. (2000). "Evidence for Recent Groundwater Seepage and Surface Runoff on Mars". Science. 288 (5475): 2330–2335. Bibcode:2000Sci...288.2330M. doi:10.1126/science.288.5475.2330. PMID 10875910.
{{cite journal}}
: Cite has empty unknown parameter:|month=
(help); Unknown parameter|coauthors=
ignored (|author=
suggested) (help) - Heldmann, Jennifer L.; et al. (May 7, 2005). "Formation of Martian gullies by the action of liquid water flowing under current Martian environmental conditions" (PDF). Journal of Geophysical Research. 110 (E5): Eo5004. Bibcode:2005JGRE..11005004H. doi:10.1029/2004JE002261. Archived from the original (– ) on December 1, 2007. Retrieved August 12, 2007.
{{cite journal}}
: Explicit use of et al. in:|last=
(help); External link in
(help); Unknown parameter|format=
|deadurl=
ignored (|url-status=
suggested) (help) 'conditions such as now occur on Mars, outside of the temperature-pressure stability regime of liquid water' … 'Liquid water is typically stable at the lowest elevations and at low latitudes on the planet because the atmospheric pressure is greater than the vapor pressure of water and surface temperatures in equatorial regions can reach 273 K for parts of the day ' - Kostama, V.-P.; Kreslavsky, M. A.; Head, J. W. (June 3, 2006). "Recent high-latitude icy mantle in the northern plains of Mars: Characteristics and ages of emplacement". Geophysical Research Letters. 33 (11): L11201. Bibcode:2006GeoRL..3311201K. doi:10.1029/2006GL025946. Retrieved August 12, 2007. 'Martian high-latitude zones are covered with a smooth, layered ice-rich mantle'
- Jpl.Nasa.Gov (December 6, 2006). "JPL news release 2006-145". Jpl.nasa.gov. Retrieved January 16, 2012.
- Malin, Michael C. (December 8, 2006). "Present-Day Impact Cratering Rate and Contemporary Gully Activity on Mars". Science. 314 (5805): 1573–1577. Bibcode:2006Sci...314.1573M. doi:10.1126/science.1135156. PMID 17158321. Retrieved September 3, 2009.
{{cite journal}}
: Unknown parameter|coauthors=
ignored (|author=
suggested) (help) - Kolb, Kelly Jean; Pelletier, Jon D.; McEwen, Alfred S. (2010). "Modeling the formation of bright slope deposits associated with gullies in Hale Crater, Mars: Implications for recent liquid water". Icarus. 205 (1): 113–137. Bibcode:2010Icar..205..113K. doi:10.1016/j.icarus.2009.09.009.
- Benison, KC; Laclair, DA (2003). "Modern and ancient extremely acid saline deposits: terrestrial analogs for martian environments?". Astrobiology. 3 (3): 609–618. Bibcode:2003AsBio...3..609B. doi:10.1089/153110703322610690. PMID 14678669.
- Benison, K; Bowen, B (2006). "Acid saline lake systems give clues about past environments and the search for life on Mars". Icarus. 183 (1): 225–229. Bibcode:2006Icar..183..225B. doi:10.1016/j.icarus.2006.02.018.
- Johnson, John (August 1, 2008). "There's water on Mars, NASA confirms". Los Angeles Times. Retrieved August 1, 2008.
- Source: Ames Research Center Posted Saturday, June 6, 2009 (June 6, 2009). "NASA Scientists Find Evidence for Liquid Water on a Frozen Early Mars | SpaceRef - Your Space Reference". SpaceRef. Retrieved January 16, 2012.
{{cite web}}
: CS1 maint: multiple names: authors list (link) CS1 maint: numeric names: authors list (link) - Fairén, Alberto G.; Davila, Alfonso F.; Gago-Duport, Luis; Amils, Ricardo; McKay, Christopher P. (2009). "Stability against freezing of aqueous solutions on early Mars". Nature. 459 (7245): 401–4. Bibcode:2009Natur.459..401F. doi:10.1038/nature07978. PMID 19458717.
{{cite journal}}
: Unknown parameter|month=
ignored (help) - Kreslavsky, M; Head, J; Marchant, D (2008). "Periods of Active Permafrost Layer Formation During the Geological History of Mars: Implication for Circum-Polar and Mid-Latitude surface Processes" (PDF). Planetary and Space Science. 56 (2): 289–302. Bibcode:2008P&SS...56..289K. doi:10.1016/j.pss.2006.02.010.
- Lobitz, B.; Wood, BL; Averner, MM; McKay, CP (2001). "Use of spacecraft data to derive regions on Mars where liquid water would be stable". Proc. Natl. Acad. Sci. U.S.A. 98 (5): 2132–7. Bibcode:2001PNAS...98.2132L. doi:10.1073/pnas.031581098. PMC 30104. PMID 11226204.
{{cite journal}}
: Unknown parameter|month=
ignored (help) - Haberle, Robert M.; McKay, Christopher P.; Schaeffer, James; Cabrol, Nathalie A.; Grin, Edmon A.; Zent, Aaron P.; Quinn, Richard (2001). "On the possibility of liquid water on present-day Mars". Journal of Geophysical Research. 106 (E10): 23317–23326. Bibcode:2001JGR...10623317H. doi:10.1029/2000JE001360.
- Nancy Atkinson (September 4, 2008). "Phoenix Probe Says Both Yes and No to Water on Mars". Universetoday.com. Retrieved January 16, 2012.
- Shiga, David (December 7, 2009). "Watery niche may foster life on Mars". New Scientist (2737).
- Mars-May-Be-Allowing-for-Life-129065.shtml
- Kostama, V.-P.; Kreslavsky, M. A.; Head, J. W. (June 3, 2006). "Recent high-latitude icy mantle in the northern plains of Mars: Characteristics and ages of emplacement". Geophysical Research Letters. 33 (11): L11201. Bibcode:2006GeoRL..3311201K. doi:10.1029/2006GL025946. Retrieved August 1, 2008.
- Fishbaugh, KE; Byrne, Shane; Herkenhoff, Kenneth E.; Kirk, Randolph L.; Fortezzo, Corey; Russell, Patrick S.; McEwen, Alfred (2010). "Evaluating the meaning of "layer" in the martian north polar layered depsoits and the impact on the climate connection" (PDF). Icarus. 205 (1): 269–282. Bibcode:2010Icar..205..269F. doi:10.1016/j.icarus.2009.04.011.
- "PSRD: Ancient Floodwaters and Seas on Mars". Psrd.hawaii.edu. July 16, 2003. Retrieved January 16, 2012.
- Carr, Michael H. (2003). "Oceans on Mars: An assessment of the observational evidence and possible fate" (PDF). Journal of Geophysical Research. 108 (E5): 5042. Bibcode:2003JGRE..108.5042C. doi:10.1029/2002JE001963.
- Zuber, Maria T. (2007). "Mars at the tipping point". Nature. 447 (7146): 785–786. Bibcode:2007Natur.447..785Z. doi:10.1038/447785a. PMID 17568733.
{{cite journal}}
: Cite has empty unknown parameters:|month=
and|coauthors=
(help) - ISBN 0-8165-1257-4
- "ESA - Mars Express - Breathtaking views of Deuteronilus Mensae on Mars". Esa.int. March 14, 2005. Retrieved January 16, 2012.
- Ohanlon, Larry (March 4, 2010). "Mars' Ice Age Revealed in Map". Discovery News.
- ^ Hauber, E.; et al. (2005). "Discovery of a flank caldera and very young glacial activity at Hecates Tholus, Mars". Nature. 434 (7031): 356–61. Bibcode:2005Natur.434..356H. doi:10.1038/nature03423. PMID 15772654.
{{cite journal}}
: Explicit use of et al. in:|author=
(help) - ^ Shean, D.; et al. (2005). "Origin and evolution of a cold-based mountain glacier on Mars: The Pavonis Mons fan-shaped deposit". Journal of Geophysical Research. 110 (E5): E05001. Bibcode:2005JGRE..11005001S. doi:10.1029/2004JE002360.
{{cite journal}}
: Explicit use of et al. in:|author=
(help) - ^ Basilevsky, A.; et al. (2006). "Geological recent tectonic, volcanic and fluvial activity on the eastern flank of the Olympus Mons volcano, Mars". Geophysical Research Letters. 33. L13201. Bibcode:2006GeoRL..3313201B. doi:10.1029/2006GL026396.
{{cite journal}}
: Explicit use of et al. in:|author=
(help) - "Fretted Terrain Valley Traverse". Hirise.lpl.arizona.edu. Retrieved January 16, 2012.
- "Jumbled Flow Patterns". Hirise.lpl.arizona.edu. Retrieved January 16, 2012.
- Berman, D.; et al. (2005). "The role of arcuate ridges and gullies in the degradation of craters in the Newton Basin region of Mars". Icarus. 178 (2): 465–86. Bibcode:2005Icar..178..465B. doi:10.1016/j.icarus.2005.05.011.
{{cite journal}}
: Explicit use of et al. in:|author=
(help) - Milliken, R.; et al. (2003). "Viscous flow features on the surface of Mars: Observations from high-resolution Mars Orbiter Camera (MOC) images". Journal of Geophysical Research. 108. E6, 5057.
{{cite journal}}
: Explicit use of et al. in:|author=
(help) - Arfstrom, J.; W. Hartmann (2005). "Martian flow features, moraine-like ridges, and gullies: Terrestrial analogs and interrelationships". Icarus. 174 (2): 321–35. Bibcode:2005Icar..174..321A. doi:10.1016/j.icarus.2004.05.026.
{{cite journal}}
: CS1 maint: multiple names: authors list (link) - Baker, V (2003). "Icy martian mysteries". Nature. 426 (6968): 779–80. doi:10.1038/426779a. PMID 14685217.
- Head, J.; et al. (2003). "Recent ice ages on Mars". Nature. 426 (6968): 797–802. doi:10.1038/nature02114. PMID 14685228.
{{cite journal}}
: Explicit use of et al. in:|author=
(help) - Mustard, J.; et al. (2001). "Evidence for recent climate change on Mars from the identification of youthful near-surface ground ice". Nature. 412 (6845): 411–4. doi:10.1038/35086515. PMID 11473309.
{{cite journal}}
: Explicit use of et al. in:|author=
(help) - Kreslavsky, M.; J. Head (2002). "Mars: Nature and evolution of young latitude-dependent water-ice-rich mantle" (PDF). Geophysical Research Letters. 29 (15). Bibcode:2002GeoRL..29o..14K. doi:10.1029/2002GL015392.
{{cite journal}}
: CS1 maint: multiple names: authors list (link) - "HiRISE | Dissected Mantled Terrain (PSP_002917_2175)". Hirise.lpl.arizona.edu. Retrieved January 16, 2012.
- Forget, F.; et al. (2006). "Formation of Glaciers on Mars by Atmospheric Precipitation at High Obliquity". Science. 311 (5759): 368–71. Bibcode:2006Sci...311..368F. doi:10.1126/science.1120335. PMID 16424337.
{{cite journal}}
: Explicit use of et al. in:|author=
(help) - Dickson, James L. (2008). "Late Amazonian glaciation at the dichotomy boundary on Mars: Evidence for glacial thickness maxima and multiple glacial phases". Geology. 36 (5): 411–4. doi:10.1130/G24382A.1.
{{cite journal}}
: Cite has empty unknown parameter:|month=
(help); Unknown parameter|coauthors=
ignored (|author=
suggested) (help) - "Origin and evolution of a cold-based tropical mountain glacier on Mars: The Pavonis Mons fan-shaped deposit" (PDF). Mars.asu.edu. Retrieved February 10, 2013.
External links
- NASA - Curiosity Rover Finds Evidence For An Ancient Streambed - September, 2012
- Images - Signs Of Water On Mars (HiRISE)
- Video (02:01) - Liquid Flowing Water Discovered on Mars - August, 2011
- Video (04:32) - Evidence: Water "Vigorously" Flowed On Mars - September, 2012
Template:Link FA Template:Link FA
Categories: