
Cochleatina canilovica, from the Late Ediacaran
A microfossil is a fossil that is generally between 0.001 mm and 1 mm in size, the visual study of which requires the use of light or electron microscopy. A fossil which can be studied with the naked eye or low-powered magnification, such as a hand lens, is referred to as a macrofossil.
Microfossils are a common feature of the geological record, from the Precambrian to the Holocene. They are most common in deposits of marine environments, but also occur in brackish water, fresh water and terrestrial sedimentary deposits. While every kingdom of life is represented in the microfossil record, the most abundant forms are protist skeletons or microbial cysts from the Chrysophyta, Pyrrhophyta, Sarcodina, acritarchs and chitinozoans, together with pollen and spores from the vascular plants.
Overview
Part of a series on |
Paleontology |
---|
![]() |
Fossils |
Natural history |
Organs and processes |
Evolution of various taxa |
Evolution |
History of paleontology |
Branches of paleontology
|
Paleontology Portal Category |
A microfossil is a descriptive term applied to fossilized plants and animals whose size is just at or below the level at which the fossil can be analyzed by the naked eye. A commonly applied cutoff point between "micro" and "macro" fossils is 1 mm. Microfossils may either be complete (or near-complete) organisms in themselves (such as the marine plankters foraminifera and coccolithophores) or component parts (such as small teeth or spores) of larger animals or plants. Microfossils are of critical importance as a reservoir of paleoclimate information, and are also commonly used by biostratigraphers to assist in the correlation of rock units.
Microfossils are found in rocks and sediments as the microscopic remains of what were once life forms such as plants, animals, fungus, protists, bacteria and archaea. Terrestrial microfossils include pollen and spores. Marine microfossils found in marine sediments are the most common microfossils. Everywhere in the oceans, microscopic protist organisms multiply prolifically, and many grow tiny skeletons which readily fossilise. These include foraminifera, dinoflagellates and radiolarians. Palaeontologists (geologists who study fossils) are interested in these microfossils because they can use them to determine how environments and climates have changed in the past, and where oil and gas can be found today.
Some microfossils are formed by colonial organisms such as Bryozoa (especially the Cheilostomata), which have relatively large colonies but are classified by fine skeletal details of the small individuals of the colony. As another example, many fossil genera of Foraminifera, which are protists are known from shells (called tests) that were as big as coins, such as the genus Nummulites.
In 2017, fossilized microorganisms, or microfossils, were discovered in hydrothermal vent precipitates in the Nuvvuagittuq Belt of Quebec, Canada that may be as old as 4.28 billion years old, the oldest record of life on Earth, suggesting "an almost instantaneous emergence of life" (in a geological time-scale), after ocean formation 4.41 billion years ago, and not long after the formation of the Earth 4.54 billion years ago. Nonetheless, life may have started even earlier, at nearly 4.5 billion years ago, as claimed by some researchers.
Index fossils
Part of a series related to |
Biomineralization |
---|
![]() |
General |
Exoskeletons (shells) |
Endoskeletons (bones) |
Teeth, scales, tusks etc |
Calcification |
Silicification |
Other forms |
Related |
Index fossils, also known as guide fossils, indicator fossils or dating fossils, are the fossilized remains or traces of particular plants or animals that are characteristic of a particular span of geologic time or environment, and can be used to identify and date the containing rocks. To be practical, index fossils must have a limited vertical time range, wide geographic distribution, and rapid evolutionary trends. Rock formations separated by great distances but containing the same index fossil species are thereby known to have both formed during the limited time that the species lived.
Index fossils were originally used to define and identify geologic units, then became a basis for defining geologic periods, and then for faunal stages and zones.
Species of microfossils such as acritarchs, chitinozoans, conodonts, dinoflagellate cysts, ostracods, pollen, spores and foraminiferans are amongst the many species have been identified as index fossils that are widely used in biostratigraphy. Different fossils work well for sediments of different ages. To work well, the fossils used must be widespread geographically, so that they can be found in many different places. They must also be short lived as a species, so that the period of time during which they could be incorporated in the sediment is relatively narrow. The longer lived the species, the poorer the stratigraphic precision, so fossils that evolve rapidly.
Often biostratigraphic correlations are based on a faunal assemblage, rather than an individual species — this allows greater precision as the time span in which all of the species in the assemblage existed together is narrower than the time spans of any of the members. Further, if only one species is present in a sample, it can mean either that (1) the strata were formed in the known fossil range of that organism; or (2) that the fossil range of the organism was incompletely known, and the strata extend the known fossil range. If the fossil is easy to preserve and easy to identify, more precise time estimating of the stratigraphic layers is possible.
Composition

Microfossils can be classified by their composition as: (a) siliceous, as in diatoms and radiolaria, (b) calcareous, as in coccoliths and foraminifera, (c) phosphatic, as in the study of some vertebrates, or (d) organic, as in the pollen and spores studied in palynology. This division focuses on differences in the mineralogical and chemical composition of microfossil remains rather than on taxonomic or ecological distinctions.
- Siliceous microfossils: Siliceous microfossils include diatoms, radiolarians, silicoflagellates, ebridians, phytoliths, some scolecodonts (worm jaws), and sponge spicules.
- Calcareous microfossils: Calcareous (CaCO3) microfossils include coccoliths, foraminifera, calcareous dinoflagellate cysts, and ostracods (seed shrimp).
- Phosphatic microfossils: Phosphatic microfossils include conodonts (tiny oral structures of an extinct chordate group), some scolecodonts (worm jaws), shark spines and teeth and other fish remains (collectively called ichthyoliths).
- Organic microfossils: The study of organic microfossils is called palynology. Organic microfossils include pollen, spores, chitinozoans (thought to be the egg cases of marine invertebrates), scolecodonts (worm jaws), acritarchs, dinoflagellate cysts, and fungal remains.
Organic-walled
Palynomorphs
Further information: Palynomorphs and KerogenPollen grain

Green: spore tetrad. Blue: spore with Y-shaped trilete mark.
Spores are about 30–35 μm across
Pollen has an outer sheath, called a sporopollenin, which affords it some resistance to the rigours of the fossilisation process that destroy weaker objects. It is produced in huge quantities. There is an extensive fossil record of pollen grains, often disassociated from their parent plant. The discipline of palynology is devoted to the study of pollen, which can be used both for biostratigraphy and to gain information about the abundance and variety of plants alive — which can itself yield important information about paleoclimates. Also, pollen analysis has been widely used for reconstructing past changes in vegetation and their associated drivers. Pollen is first found in the fossil record in the late Devonian period, but at that time it is indistinguishable from spores. It increases in abundance until the present day.
Plant spores
See also: CryptosporeA spore is a unit of sexual or asexual reproduction that may be adapted for dispersal and for survival, often for extended periods of time, in unfavourable conditions. Spores form part of the life cycles of many plants, algae, fungi and protozoa. Bacterial spores are not part of a sexual cycle but are resistant structures used for survival under unfavourable conditions.
Fungal spores
Chitinozoa

Chitinozoa are a taxon of flask-shaped, organic walled marine microfossils produced by an as yet unknown organism.
Common from the Ordovician to Devonian periods (i.e. the mid-Paleozoic), the millimetre-scale organisms are abundant in almost all types of marine sediment across the globe. This wide distribution, and their rapid pace of evolution, makes them valuable biostratigraphic markers.
Their bizarre form has made classification and ecological reconstruction difficult. Since their discovery in 1931, suggestions of protist, plant, and fungal affinities have all been entertained. The organisms have been better understood as improvements in microscopy facilitated the study of their fine structure, and it has been suggested that they represent either the eggs or juvenile stage of a marine animal. However, recent research has suggested that they represent the test of a group of protists with uncertain affinities.
The ecology of chitinozoa is also open to speculation; some may have floated in the water column, where others may have attached themselves to other organisms. Most species were particular about their living conditions, and tend to be most common in specific paleoenvironments. Their abundance also varied with the seasons.
Acritarchs

c. 570–609 mya
Acritarchs, Greek for confused origins, are organic-walled microfossils, known from about 2,000 million years ago to the present. Acritarchs are not a specific biological taxon, but rather a group with uncertain or unknown affinities. Most commonly they are composed of thermally altered acid insoluble carbon compounds (kerogen). While the classification of acritarchs into form genera is entirely artificial, it is not without merit, as the form taxa show traits similar to those of genuine taxa — for example the 'explosion' in the Cambrian and the mass extinction at the end of the Permian.
Acritarch diversity reflects major ecological events such as the appearance of predation and the Cambrian explosion. Precambrian marine diversity was dominated by acritarchs. They underwent a boom around 1,000 million years ago, increasing in abundance, diversity, size, complexity of shape, and especially size and number of spines. Their increasingly spiny forms in the last 1 billion years may indicate an increased need for defence against predation.
Acritarchs may include the remains of a wide range of quite different kinds of organisms—ranging from the egg cases of small metazoans to resting cysts of many kinds of chlorophyta (green algae). It is likely that most acritarch species from the Paleozoic represent various stages of the life cycle of algae that were ancestral to the dinoflagellates. The nature of the organisms associated with older acritarchs is generally not well understood, though many are probably related to unicellular marine algae. In theory, when the biological source (taxon) of an acritarch does become known, that particular microfossil is removed from the acritarchs and classified with its proper group.
Acritarchs were most likely eukaryotes. While archaea, bacteria and cyanobacteria (prokaryotes) usually produce simple fossils of a very small size, eukaryotic unicellular fossils are usually larger and more complex, with external morphological projections and ornamentation such as spines and hairs that only eukaryotes can produce; as most acritarchs have external projections (e.g., hair, spines, thick cell membranes, etc.), they are predominantly eukaryotes, although simple eukaryote acritarchs also exist.
Acritarchs are found in sedimentary rocks from the present back into the Archean. They are typically isolated from siliciclastic sedimentary rocks using hydrofluoric acid but are occasionally extracted from carbonate-rich rocks. They are excellent candidates for index fossils used for dating rock formations in the Paleozoic Era and when other fossils are not available. Because most acritarchs are thought to be marine (pre-Triassic), they are also useful for palaeoenvironmental interpretation. The Archean and earliest Proterozoic microfossils termed "acritarchs" may actually be prokaryotes. The earliest eukaryotic acritarchs known (as of 2020) are from between 1950 and 2150 million years ago.
Recent application of atomic force microscopy, confocal microscopy, Raman spectroscopy, and other analytic techniques to the study of the ultrastructure, life history, and systematic affinities of mineralized, but originally organic-walled microfossils, have shown some acritarchs are fossilized microalgae. In the end, it may well be, as Moczydłowska et al. suggested in 2011, that many acritarchs will, in fact, turn out to be algae.

Archean cells
See also: Archean life in the Barberton Greenstone BeltCells can be preserved in the rock record because their cell walls are made of proteins which convert to the organic material kerogen as the cell breaks down after death. Kerogen is insoluble in mineral acids, bases, and organic solvents. Over time, it is mineralised into graphite or graphite-like carbon, or degrades into oil and gas hydrocarbons. There are three main types of cell morphologies. Though there is no established range of sizes for each type, spheroid microfossils can be as small as about 8 micrometres, filamentous microfossils have diameters typically less than 5 micrometres and have a length that can range from tens of micrometres to 100 micrometres, and spindle-like microfossils can be as long as 50 micrometres.
Mineralised
Further information: Shelled protistsSiliceous
Siliceous ooze is a type of biogenic pelagic sediment located on the deep ocean floor. Siliceous oozes are the least common of the deep sea sediments, and make up approximately 15% of the ocean floor. Oozes are defined as sediments which contain at least 30% skeletal remains of pelagic microorganisms. Siliceous oozes are largely composed of the silica based skeletons of microscopic marine organisms such as diatoms and radiolarians. Other components of siliceous oozes near continental margins may include terrestrially derived silica particles and sponge spicules. Siliceous oozes are composed of skeletons made from opal silica Si(O2), as opposed to calcareous oozes, which are made from skeletons of calcium carbonate organisms (i.e. coccolithophores). Silica (Si) is a bioessential element and is efficiently recycled in the marine environment through the silica cycle. Distance from land masses, water depth and ocean fertility are all factors that affect the opal silica content in seawater and the presence of siliceous oozes.
Siliceous ooze | |||||||
---|---|---|---|---|---|---|---|
mineral forms |
protist involved |
name of skeleton | typical size | ||||
SiO2 silica quartz glass opal chert |
diatom | ![]() |
frustule | 0.002 to 0.2 mm | ![]() |
diatom microfossil from 40 million years ago | |
radiolarian | ![]() |
test or shell | 0.1 to 0.2 mm | ![]() |
elaborate silica shell of a radiolarian |
-
Diatomaceous earth is a soft, siliceous, sedimentary rock made up of microfossils in the form of the frustules (shells) of centric and pennate diatoms (click to magnify)
-
Centric diatom
(radial symmetry) -
Pennate diatom
(bilateral symmetry)



Cornus controversa • scale bar 20 μm
Phytoliths (Greek for plant stones) are rigid, microscopic structures made of silica, found in some plant tissues and persisting after the decay of the plant. These plants take up silica from the soil, whereupon it is deposited within different intracellular and extracellular structures of the plant. Phytoliths come in varying shapes and sizes. The term "phytolith" is sometimes used to refer to all mineral secretions by plants, but more commonly refers to siliceous plant remains.
Calcareous
The term calcareous can be applied to a fossil, sediment, or sedimentary rock which is formed from, or contains a high proportion of, calcium carbonate in the form of calcite or aragonite. Calcareous sediments (limestone) are usually deposited in shallow water near land, since the carbonate is precipitated by marine organisms that need land-derived nutrients. Generally speaking, the farther from land sediments fall, the less calcareous they are. Some areas can have interbedded calcareous sediments due to storms, or changes in ocean currents. Calcareous ooze is a form of calcium carbonate derived from planktonic organisms that accumulates on the sea floor. This can only occur if the ocean is shallower than the carbonate compensation depth. Below this depth, calcium carbonate begins to dissolve in the ocean, and only non-calcareous sediments are stable, such as siliceous ooze or pelagic red clay.
Calcareous ooze | |||||||
---|---|---|---|---|---|---|---|
mineral forms |
protist involved |
name of skeleton | typical size | ||||
CaCO3 calcite aragonite limestone marble chalk |
foraminiferan | ![]() |
test or shell | many under 1 mm | ![]() |
Calcified test of a planktic foraminiferan. There are about 10,000 living species of foraminiferans | |
coccolithophore | ![]() |
coccoliths | under 0.1 mm | ![]() |
Coccolithophores are the largest global source of biogenic calcium carbonate, and significantly contribute to the global carbon cycle. They are the main constituent of chalk deposits such as the white cliffs of Dover. |
-
Calcareous microfossils from marine sediment consisting mainly of star-shaped discoaster with a sprinkling of coccoliths
-
Illustration of a Globigerina ooze
-
Shells (tests), usually made of calcium carbonate, from a foraminiferal ooze on the deep ocean floor


Scale bar: 100 μm
Ostracods

Ostracods are widespread crustaceans, generally small, sometimes known as seed shrimps. They are flattened from side to side and protected with a calcareous or chitinous bivalve-like shell. There are about 70,000 known species, 13,000 of which are extant. Ostracods are typically about 1 mm (0.039 in) in size, though they can range from 0.2 to 30 mm (0.008 to 1.181 in), with some species such as Gigantocypris being too large to be regarded as microfossils.
Conodonts

Conodonts (cone tooth in Greek) are tiny, extinct jawless fish that resemble eels. For many years, they were known only from tooth-like microfossils found in isolation and now called conodont elements. The evolution of mineralized tissues has been a puzzle for more than a century. It has been hypothesized that the first mechanism of chordate tissue mineralization began either in the oral skeleton of conodont or the dermal skeleton of early agnathans. Conodont elements are made of a phosphatic mineral, hydroxylapatite.
The element array constituted a feeding apparatus that is radically different from the jaws of modern animals. They are now termed "conodont elements" to avoid confusion. The three forms of teeth (i.e., coniform cones, ramiform bars, and pectiniform platforms) probably performed different functions. For many years, conodonts were known only from enigmatic tooth-like microfossils (200 micrometres to 5 millimetres in length) which occur commonly, but not always in isolation, and were not associated with any other fossil.
Conodonts are globally widespread in sediments.Their many forms are considered index fossils, fossils used to define and identify geological periods and date strata. Conodonts elements can be used to estimate the temperatures rocks have been exposed to, which allows the thermal maturation levels of sedimentary rocks to be determined, which is important for hydrocarbon exploration. Conodont teeth are the earliest vertebrate teeth found in the fossil record, and some conodont teeth are the sharpest that have ever been recorded.
Scolecodonts
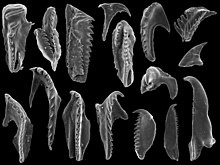
from the Ordovician and Silurian
Scolecodonts (worm jaws in Latin) are tiny jaws of polychaete annelids of the order Eunicida - a diverse and abundant group of worms which has been inhabiting different marine environments in the past 500 million years. Composed of highly resistant organic substance, the scolecodonts are frequently found as fossils from the rocks as old as the late Cambrian. Since the worms themselves were soft-bodied and hence extremely rarely preserved in the fossil record, their jaws constitute the main evidence of polychaetes in the geological past, and the only way to restore the evolution of this important group of animals. Small size of scolecodonts, usually less than 1 mm, puts them into a microfossil category. They are common by-product of conodont, chitinozoan and acritarch samples, but sometimes they occur in the sediments where other fossils are very rare or absent.
Cloudinids
The cloudinids were an early metazoan family that lived in the late Ediacaran period about 550 million years ago, and became extinct at the base of the Cambrian. They formed small millimetre size conical fossils consisting of calcareous cones nested within one another; the appearance of the organism itself remains unknown. The name Cloudina honors Preston Cloud. Fossils consist of a series of stacked vase-like calcite tubes, whose original mineral composition is unknown, Cloudinids comprise two genera: Cloudina itself is mineralized, whereas Conotubus is at best weakly mineralized, whilst sharing the same "funnel-in-funnel" construction.
Cloudinids had a wide geographic range, reflected in the present distribution of localities in which their fossils are found, and are an abundant component of some deposits. Cloudina is usually found in association with microbial stromatolites, which are limited to shallow water, and it has been suggested that cloudinids lived embedded in the microbial mats, growing new cones to avoid being buried by silt. However no specimens have been found embedded in mats, and their mode of life is still an unresolved question.
The classification of the cloudinids has proved difficult: they were initially regarded as polychaete worms, and then as coral-like cnidarians on the basis of what look like buds on some specimens. Current scientific opinion is divided between classifying them as polychaetes and regarding it as unsafe to classify them as members of any broader grouping. In 2020, a new study showed the presence of Nephrozoan type guts, the oldest on record, supporting the bilaterian interpretation.
Cloudinids are important in the history of animal evolution for two reasons. They are among the earliest and most abundant of the small shelly fossils with mineralized skeletons, and therefore feature in the debate about why such skeletons first appeared in the Late Ediacaran. The most widely supported answer is that their shells are a defense against predators, as some Cloudina specimens from China bear the marks of multiple attacks, which suggests they survived at least a few of them. The holes made by predators are approximately proportional to the size of the Cloudina specimens, and Sinotubulites fossils, which are often found in the same beds, have so far shown no such holes. These two points suggest that predators attacked in a selective manner, and the evolutionary arms race which this indicates is commonly cited as a cause of the Cambrian explosion of animal diversity and complexity.
Dinoflagellate cysts

as drawn by Ehrenberg in 1837
Some dinoflagellates produce resting stages, called dinoflagellate cysts or dinocysts, as part of their lifecycles. Dinoflagellates are mainly represented in the fossil record by these dinocysts, typically 15 to 100 micrometres in diameter, which accumulate in sediments as microfossils. Organic-walled dinocysts have resistant cell walls made out of dinosporin. There are also calcareous dinoflagellate cysts and siliceous dinoflagellate cysts.
Dinocysts are produced by a proportion of dinoflagellates as a dormant, zygotic stage of their lifecycle. These dinocyst stages are known to occur in 84 of the 350 described freshwater dinoflagellate species, and in about 10% of the known marine species. Dinocysts have a long geological record with geochemical markers suggest a presence that goes back to the Early Cambrian.
Sponge spicules

Spicules are structural elements found in most sponges. They provide structural support and deter predators. The meshing of many spicules serves as the sponge's skeleton, providing structural support and defense against predators.
Smaller, microscopic spicules can become microfossils, and are referred to as microscleres. Larger spicules visible to the naked eye are called megascleres. Spicule can be calcareous, siliceous, or composed of spongin. They are found in a range of symmetry types.

Freshwater sediments
See also: PaleolimnologyMarine sediments

Within each colored area, the type of material shown is what dominates, although other materials are also likely to be present.
For further information, see here

Sediments at the bottom of the ocean have two main origins, terrigenous and biogenous.
Terrigenous sediments account for about 45% of the total marine sediment, and originate in the erosion of rocks on land, transported by rivers and land runoff, windborne dust, volcanoes, or grinding by glaciers.
Biogenous
Biogenous sediments account for the other 55% of the total sediment, and originate in the skeletal remains of marine protists (single-celled plankton and benthos microorganisms). Much smaller amounts of precipitated minerals and meteoric dust can also be present. Ooze, in the context of a marine sediment, does not refer to the consistency of the sediment but to its biological origin. The term ooze was originally used by John Murray, the "father of modern oceanography", who proposed the term radiolarian ooze for the silica deposits of radiolarian shells brought to the surface during the Challenger expedition. A biogenic ooze is a pelagic sediment containing at least 30 per cent from the skeletal remains of marine organisms.
Lithified
Further information: sedimentary rock-
Opal can include microfossil diatoms, radiolarians, silicoflagellates and ebridians
-
Marble can contain microfossil foraminiferans, coccolithophores, calcareous nannoplankton and algae, ostracodes, pteropods, calpionellids and bryozoa



Micropaleontology
Main article: MicropaleontologyThe study of microfossils is called micropaleontology. In micropaleontology, what would otherwise be distinct categories are grouped together based solely on their size, including microscopic organisms and minute parts of larger organisms. Numerous sediments have microfossils, which serve as significant biostratigraphic, paleoenvironmental, and paleoceanographic markers. Their widespread presence around the world and physical toughness makes microfossils important for biostratigraphy, while the manner in which they have reacted to environmental changes makes them helpful when reconstructing past environments.
See also
- Biosignature
- Biostratigraphy
- Chemostratigraphy
- Gunflint microfossils
- Macrofossil
- Protists in the fossil record
- Protist shell
- Scale microfossils
- Small carbonaceous fossil
References
- Slater, Ben J.; Harvey, Thomas H. P.; Bekker, Andrey; Butterfield, Nicholas J. (2020). "Cochleatina: an enigmatic Ediacaran–Cambrian survivor among small carbonaceous fossils (SCFs)". Palaeontology. 63 (5): 733–752. Bibcode:2020Palgy..63..733S. doi:10.1111/pala.12484. ISSN 1475-4983.
- Drewes, Charlie. "Discovering Devonian Microfossils". Iowa State University. Retrieved 4 March 2017.
- Campbell, Hamish (12 Jun 2006) "Fossils - Microfossils", Te Ara - the Encyclopedia of New Zealand". Accessed 11 May 2021.
- Dodd, Matthew S.; Papineau, Dominic; Grenne, Tor; slack, John F.; Rittner, Martin; Pirajno, Franco; O'Neil, Jonathan; Little, Crispin T. S. (2 March 2017). "Evidence for early life in Earth's oldest hydrothermal vent precipitates" (PDF). Nature. 543 (7643): 60–64. Bibcode:2017Natur.543...60D. doi:10.1038/nature21377. PMID 28252057.
- Zimmer, Carl (1 March 2017). "Scientists Say Canadian Bacteria Fossils May Be Earth's Oldest". New York Times. Retrieved 2 March 2017.
- Ghosh, Pallab (1 March 2017). "Earliest evidence of life on Earth 'found". BBC News. Retrieved 2 March 2017.
- Dunham, Will (1 March 2017). "Canadian bacteria-like fossils called oldest evidence of life". Reuters. Archived from the original on 2 March 2017. Retrieved 1 March 2017.
- Staff (20 August 2018). "A timescale for the origin and evolution of all of life on Earth". Phys.org. Retrieved 20 August 2018.
- Betts, Holly C.; Putick, Mark N.; Clark, James W.; Williams, Tom A.; Donoghue, Philip C.J.; Pisani, Davide (20 August 2018). "Integrated genomic and fossil evidence illuminates life's early evolution and eukaryote origin". Nature. 2 (10): 1556–1562. doi:10.1038/s41559-018-0644-x. PMC 6152910. PMID 30127539.
- Gray, J.; Chaloner, W. G.; Westoll, T. S. (1985). "The Microfossil Record of Early Land Plants: Advances in Understanding of Early Terrestrialization, 1970–1984". Philosophical Transactions of the Royal Society B. 309 (1138): 167–195. Bibcode:1985RSPTB.309..167G. doi:10.1098/rstb.1985.0077. JSTOR 2396358.
- Franco-Gaviria, Felipe; et al. (2018). "The human impact imprint on modern pollen spectra of the Mayan lands" (PDF). Boletín de la Sociedad Geológica Mexicana. 70 (1): 61–78. doi:10.18268/BSGM2018v70n1a4.
- ^ Traverse, Alfred (2007). "Devonian Palynology". Paleopalynology. Topics in Geobiology, 28. Vol. 28. Dordrecht: Springer. pp. 199–227. doi:10.1007/978-1-4020-5610-9_8. ISBN 978-1-4020-6684-9.
- Wang, De-Ming; Meng, Mei-Cen; Guo, Yun (2016). "Pollen Organ Telangiopsis sp. of Late Devonian Seed Plant and Associated Vegetative Frond". PLOS ONE. 11 (1): e0147984. Bibcode:2016PLoSO..1147984W. doi:10.1371/journal.pone.0147984. PMC 4725745. PMID 26808271.
- "Tree of Life Web Project". Archived from the original on 5 February 2018. Retrieved 5 February 2018.
- Gary Lee Mullins (2000). "A chitinozoan morphological lineage and its importance in Lower Silurian stratigraphy". Palaeontology. 43 (2): 359–373. Bibcode:2000Palgy..43..359M. doi:10.1111/1475-4983.00131.
- Jansonius, J.; Jenkins, W.A.M. (1978). "Chitinozoa". Introduction to marine micropaleontology. Elsevier, New York. pp. 341–357. ISBN 0-444-00267-7.
- Gabbott, S.E.; Aldridge, R.J.; Theron, J.N. (1998). "Chitinozoan chains and cocoons from the Upper Ordovician Soom Shale lagerstatte, South Africa; implications for affinity". Journal of the Geological Society. 155 (3): 447–452. Bibcode:1998JGSoc.155..447G. doi:10.1144/gsjgs.155.3.0447. S2CID 129236534.
- Liang, Yan; Hints, Olle; Tang, Peng; Cai, Chenyang; Goldman, Daniel; Nõlvak, Jaak; Tihelka, Erik; Pang, Ke; Bernardo, Joseph; Wang, Wenhui (1 December 2020). "Fossilized reproductive modes reveal a protistan affinity of Chitinozoa". Geology. 48 (12): 1200–1204. Bibcode:2020Geo....48.1200L. doi:10.1130/G47865.1. ISSN 0091-7613.
- Cunningham, John A.; Vargas, Kelly; Yin, Zongjun; Bengtson, Stefan; Donoghue, Philip C. J. (2017). "The Weng'an Biota (Doushantuo Formation): An Ediacaran window on soft-bodied and multicellular microorganisms". Journal of the Geological Society. 174 (5): 793–802. Bibcode:2017JGSoc.174..793C. doi:10.1144/jgs2016-142. hdl:1983/d874148a-f20e-498a-97d2-379b3feaa18a.
- definition of acritarch at dictionary.com
- Evitt, W. R. (1963). "A Discussion and Proposals Concerning Fossil Dinoflagellates, Hystrichospheres, and Acritarchs, Ii". Proceedings of the National Academy of Sciences. 49 (3): 298–302. Bibcode:1963PNAS...49..298E. doi:10.1073/pnas.49.3.298. PMC 299818. PMID 16591055.
- Martin, Francine (1993). "Acritarchsa Review". Biological Reviews. 68 (4): 475–537. doi:10.1111/j.1469-185X.1993.tb01241.x. S2CID 221527533.
- Colbath, G.Kent; Grenfell, Hugh R. (1995). "Review of biological affinities of Paleozoic acid-resistant, organic-walled eukaryotic algal microfossils (Including "acritarchs")". Review of Palaeobotany and Palynology. 86 (3–4): 287–314. Bibcode:1995RPaPa..86..287C. doi:10.1016/0034-6667(94)00148-D.
- Bengtson, S. (2002). "Origins and early evolution of predation". In Kowalewski, M.; Kelley, P.H. (eds.). The fossil record of predation. The Paleontological Society Papers 8 (Free full text). The Paleontological Society. pp. 289–317. Retrieved 1 December 2007.
- Colbath, G.Kent; Grenfell, Hugh R. (1995). "Review of biological affinities of Paleozoic acid-resistant, organic-walled eukaryotic algal microfossils (including "acritarchs")". Review of Palaeobotany and Palynology. 86 (3–4): 287–314. Bibcode:1995RPaPa..86..287C. doi:10.1016/0034-6667(94)00148-d. ISSN 0034-6667.
- Buick, R. . (2010). "Early life: Ancient acritarchs". Nature. 463 (7283): 885–886. Bibcode:2010Natur.463..885B. doi:10.1038/463885a. PMID 20164911.
- "MONTENARI, M. & LEPPIG, U. (2003): The Acritarcha: their classification morphology, ultrastructure and palaeoecological/palaeogeographical distribution". Paläontologische Zeitschrift. 77: 173–194. 2003. doi:10.1007/bf03004567. S2CID 127238427.
- Yin, Leiming (February 2020). "Microfossils from the Paleoproterozoic Hutuo Group, Shanxi, North China: Early evidence for eukaryotic metabolism". Precambrian Research. 342: 105650. Bibcode:2020PreR..342j5650Y. doi:10.1016/j.precamres.2020.105650.
- Kempe, A.; Schopf, J. W.; Altermann, W.; Kudryavtsev, A. B.; Heckl, W. M. (2002). "Atomic force microscopy of Precambrian microscopic fossils". Proceedings of the National Academy of Sciences. 99 (14): 9117–9120. Bibcode:2002PNAS...99.9117K. doi:10.1073/pnas.142310299. PMC 123103. PMID 12089337.
- Kempe, A.; Wirth, R.; Altermann, W.; Stark, R.; Schopf, J.; Heckl, W. (2005). "Focussed ion beam preparation and in situ nanoscopic study of Precambrian acritarchs". Precambrian Research. 140 (1–2): 36–54. Bibcode:2005PreR..140...36K. doi:10.1016/j.precamres.2005.07.002.
- Marshall, C.; Javaux, E.; Knoll, A.; Walter, M. (2005). "Combined micro-Fourier transform infrared (FTIR) spectroscopy and micro-Raman spectroscopy of Proterozoic acritarchs: A new approach to Palaeobiology". Precambrian Research. 138 (3–4): 208–224. Bibcode:2005PreR..138..208M. doi:10.1016/j.precamres.2005.05.006.
- Kaźmierczak, Józef; Kremer, Barbara (2009). "Spore-Like Bodies in Some Early Paleozoic Acritarchs: Clues to Chlorococcalean Affinities". Acta Palaeontologica Polonica. 54 (3): 541–551. doi:10.4202/app.2008.0060.
- Schopf, J. William; Kudryavtsev, Anatoliy B.; Sergeev, Vladimir N. (2010). "Confocal laser scanning microscopy and Raman imagery of the late Neoproterozoic Chichkan microbiota of South Kazakhstan". Journal of Paleontology. 84 (3): 402–416. Bibcode:2010JPal...84..402S. doi:10.1666/09-134.1. S2CID 130041483.
- Moczydłowska, Małgorzata; Landing, ED; Zang, Wenlong; Palacios, Teodoro (2011). "Proterozoic phytoplankton and timing of Chlorophyte algae origins". Palaeontology. 54 (4): 721–733. Bibcode:2011Palgy..54..721M. doi:10.1111/j.1475-4983.2011.01054.x.
- Chamberlain, John; Chamberlain, Rebecca; Brown, James (2016). "A Mineralized Alga and Acritarch Dominated Microbiota from the Tully Formation (Givetian) of Pennsylvania, USA". Geosciences. 6 (4): 57. Bibcode:2016Geosc...6...57C. doi:10.3390/geosciences6040057.
Material was copied from this source, which is available under a Creative Commons Attribution 4.0 International License.
- Philp, R.P.; Calvin, M. (1976). "Possible origin for insoluble organic (kerogen) debris in sediments from insoluble cell-wall materials of algae and bacteria". Nature. 262 (5564): 134–136. Bibcode:1976Natur.262..134P. doi:10.1038/262134a0. S2CID 42212699.
- Tegelaar, E.W.; deLeeuw, J.W.; Derenne, S.; Largeau, C. (1989). "A reappraisal of kerogen formation". Geochimica et Cosmochimica Acta. 53 (11): 3103–3106. Bibcode:1989GeCoA..53.3103T. doi:10.1016/0016-7037(89)90191-9.
- Walsh, M. (1991). "Microfossils and possible microfossils from the early Archean Onverwacht Group, Barberton mountain land, South Africa". Precambrian Research. 54 (2–4): 271–293. doi:10.1016/0301-9268(92)90074-X. PMID 11540926.
- Oehler, D.Z.; Robert, F.; Mostefaoui, S.; Meibom, A.; Selo, M.; McKay, D.S. (2006). "Chemical Mapping of Proterozoic Organic Matter at Submicron Spatial Resolution". Astrobiology. 6 (6): 838–850. Bibcode:2006AsBio...6..838O. doi:10.1089/ast.2006.6.838. hdl:2060/20060028086. PMID 17155884.
- Mulder, Thierry; Hüneke, Heiko; Van Loon, A.J. (2011), "Progress in Deep-Sea Sedimentology", Deep-Sea Sediments, Elsevier, pp. 1–24, doi:10.1016/b978-0-444-53000-4.00001-9, ISBN 9780444530004
- Bohrmann, Gerhard; Abelmann, Andrea; Gersonde, Rainer; Hubberten, Hans; Kuhn, Gerhard (1994). "Pure siliceous ooze, a diagenetic environment for early chert formation". Geology. 22 (3): 207. Bibcode:1994Geo....22..207B. doi:10.1130/0091-7613(1994)022<0207:psoade>2.3.co;2.
- DeMaster, David J. (October 1981). "The supply and accumulation of silica in the marine environment". Geochimica et Cosmochimica Acta. 45 (10): 1715–1732. Bibcode:1981GeCoA..45.1715D. doi:10.1016/0016-7037(81)90006-5.
- Hasle, Grethe R.; Syvertsen, Erik E.; Steidinger, Karen A.; Tangen, Karl (25 January 1996). "Marine Diatoms". In Tomas, Carmelo R. (ed.). Identifying Marine Diatoms and Dinoflagellates. Academic Press. pp. 5–385. ISBN 978-0-08-053441-1. Retrieved 13 November 2013.
- Ge, Yong; Lu, Houyuan; Wang, Can; Gao, Xing (2020). "Phytoliths in selected broad-leaved trees in China". Scientific Reports. 10 (1): 15577. Bibcode:2020NatSR..1015577G. doi:10.1038/s41598-020-72547-w. PMC 7512002. PMID 32968165.
- Piperno, Dolores R. (2006). Phytoliths: A Comprehensive Guide for Archaeologists and Paleoecologists. AltaMira Press ISBN 0759103852.
- Ald, S.M.; et al. (2007). "Diversity, Nomenclature, and Taxonomy of Protists". Syst. Biol. 56 (4): 684–689. doi:10.1080/10635150701494127. PMID 17661235.
- Moheimani, N.R.; Webb, J.P.; Borowitzka, M.A. (2012), "Bioremediation and other potential applications of coccolithophorid algae: A review. . Bioremediation and other potential applications of coccolithophorid algae: A review", Algal Research, 1 (2): 120–133, doi:10.1016/j.algal.2012.06.002
- Taylor, A.R.; Chrachri, A.; Wheeler, G.; Goddard, H.; Brownlee, C. (2011). "A voltage-gated H+ channel underlying pH homeostasis in calcifying coccolithophores". PLOS Biology. 9 (6): e1001085. doi:10.1371/journal.pbio.1001085. PMC 3119654. PMID 21713028.
- Fox, Lyndsey R.; Stukins, Stephen; Hill, Tom; Bailey, Haydon W. (2018). "New species of Mesozoic benthic foraminifera from the former British Petroleum micropalaeontology collection". Journal of Micropalaeontology. 37 (1): 395–401. Bibcode:2018JMicP..37..395F. doi:10.5194/jm-37-395-2018. hdl:10141/622407.
- Castellani, Christopher; Maas, Andreas; Eriksson, Mats E.; Haug, Joachim T.; Haug, Carolin; Waloszek, Dieter (2018). "First record of Cyanobacteria in Cambrian Orsten deposits of Sweden". Palaeontology. 61 (6): 855–880. Bibcode:2018Palgy..61..855C. doi:10.1111/pala.12374.
- Richard C. Brusca & Gary J. Brusca (2003). Invertebrates (2nd ed.). Sinauer Associates. ISBN 978-0-87893-097-5.
- ^ Shubin, Neil (2009). Your Inner Fish: A Journey into the 3.5 Billion Year History of the Human Body (reprint ed.). New York: Pantheon Books. pp. 85–86. ISBN 9780307277459.
- Trotter, Julie A. (2006). "Chemical systematics of conodont apatite determined by laser ablation ICPMS". Chemical Geology. 233 (3–4): 196–216. Bibcode:2006ChGeo.233..196T. doi:10.1016/j.chemgeo.2006.03.004.
- MIRACLE. "Conodonts". Retrieved 26 August 2014.
- Study of microfossils maps extreme global warming and environmental change Phys.org, 7 August 2019.
- Wu, Kui; Tian, Li; Liang, Lei; Metcalfe, Ian; Chu, Daoliang; Tong, Jinnan (2019). "Recurrent biotic rebounds during the Early Triassic: Biostratigraphy and temporal size variation of conodonts from the Nanpanjiang Basin, South China". Journal of the Geological Society. 176 (6): 1232–1246. Bibcode:2019JGSoc.176.1232W. doi:10.1144/jgs2019-065. S2CID 202181855.
- Scientists Discover Sharpest Teeth in History Sci-News.com, 20 March 2012.
- Jones, David; Evans, Alistair R.; Siu, Karen K. W.; Rayfield, Emily J.; Donoghue, Philip C. J. (2012). "The sharpest tools in the box? Quantitative analysis of conodont element functional morphology". Proceedings of the Royal Society B: Biological Sciences. 279 (1739): 2849–2854. doi:10.1098/rspb.2012.0147. PMC 3367778. PMID 22418253.
- ^ Hints, Olle (2016) Scolecodonts — jaws of polychaete annelids Institute of Geology at Tallinn University of Technology.
Material was copied from this source, which is available under a Creative Commons Attribution 4.0 International License.
- Joel, Lucas (10 January 2020). "Fossil Reveals Earth's Oldest Known Animal Guts - The find in a Nevada desert revealed an intestine inside a creature that looks like a worm made of a stack of ice cream cones". The New York Times. Retrieved 10 January 2020.
- ^ Schiffbauer, James D.; et al. (10 January 2020). "Discovery of bilaterian-type through-guts in cloudinomorphs from the terminal Ediacaran Period". Nature Communications. 11 (205): 205. Bibcode:2020NatCo..11..205S. doi:10.1038/s41467-019-13882-z. PMC 6954273. PMID 31924764.
- Yang, Ben; Steiner, Michael; Zhu, Maoyan; Li, Guoxiang; Liu, Jianni; Liu, Pengju (2016). "Transitional Ediacaran–Cambrian small skeletal fossil assemblages from South China and Kazakhstan: Implications for chronostratigraphy and metazoan evolution". Precambrian Research. 285: 202–215. Bibcode:2016PreR..285..202Y. doi:10.1016/j.precamres.2016.09.016.
- Germs, G.J.B. (October 1972). "New shelly fossils from Nama Group, South West Africa". American Journal of Science. 272 (8): 752–761. Bibcode:1972AmJS..272..752G. doi:10.2475/ajs.272.8.752.
- Porter, S.M. (1 June 2007). "Seawater Chemistry and Early Carbonate Biomineralization". Science. 316 (5829): 1302. Bibcode:2007Sci...316.1302P. doi:10.1126/science.1137284. PMID 17540895. S2CID 27418253.
- Smith, E.F.; Nelson, L.L.; Strange, M.A.; Eyster, A.E.; Rowland, S.M.; Schrag, D.P.; MacDonald, F.A. (2016). "The end of the Ediacaran: Two new exceptionally preserved body fossil assemblages from Mount Dunfee, Nevada, USA". Geology. 44 (11): 911. Bibcode:2016Geo....44..911S. doi:10.1130/G38157.1.
- Mertens KN, Rengefors K, Moestrup Ø, Ellegaard M (2012). "A review of recent freshwater dinoflagellate cysts: Taxonomy, phylogeny, ecology and palaeocology". Phycologia. 51 (6): 612–619. doi:10.2216/11-89.1. S2CID 86845462.
- Bravo I, Figueroa RI (January 2014). "Towards an Ecological Understanding of Dinoflagellate Cyst Functions". Microorganisms. 2 (1): 11–32. doi:10.3390/microorganisms2010011. PMC 5029505. PMID 27694774.
- Moldowan JM, Talyzina NM (August 1998). "Biogeochemical evidence for dinoflagellate ancestors in the Early Cambrian". Science. 281 (5380): 1168–70. Bibcode:1998Sci...281.1168M. doi:10.1126/science.281.5380.1168. PMID 9712575.
- Jones, Adam C.; Blum, James E.; Pawlik, Joseph R. (8 August 2005). "Testing for defensive synergy in Caribbean sponges: Bad taste or glass spicules?". Journal of Experimental Marine Biology and Ecology. 322 (1): 67–81. doi:10.1016/j.jembe.2005.02.009. ISSN 0022-0981. S2CID 85614908.
- Thomson, Charles Wyville (2014) Voyage of the Challenger : The Atlantic Cambridge University Press, page235. ISBN 9781108074759.
- ^ Haq B.U. and Boersma A. (Eds.) (1998) Introduction to Marine Micropaleontology Elsevier. ISBN 9780080534961
- Wierer, U.; Arrighi, S.; Bertola, S.; Kaufmann, G.; Baumgarten, B.; Pedrotti, A.; Pernter, P.; Pelegrin, J. (2018). "The Iceman's lithic toolkit: Raw material, technology, typology and use". PLOS ONE. 13 (6): e0198292. Bibcode:2018PLoSO..1398292W. doi:10.1371/journal.pone.0198292. PMC 6010222. PMID 29924811.
- Armstrong, Howard; Brasier, Martin (2013). Microfossils. New York, NY. ISBN 978-1-118-68545-7. OCLC 904814387.
{{cite book}}
: CS1 maint: location missing publisher (link) - Martin, Ronald E. (2000). Environmental Micropaleontology : the Application of Microfossils to Environmental Geology. New York. ISBN 978-1-4615-4167-7. OCLC 840285428.
{{cite book}}
: CS1 maint: location missing publisher (link)
Other sources
- De Wever, Patrick (2020). Marvelous microfossils : creators, timekeepers, architects. Baltimore. ISBN 978-1-4214-3674-6. OCLC 1148175375.
{{cite book}}
: CS1 maint: location missing publisher (link)