Part of a series on |
Plankton |
---|
![]() |
Trophic mode |
By size |
By taxonomy |
By habitat |
Other types |
Blooms |
Related topics |
Bacterioplankton refers to the bacterial component of the plankton that drifts in the water column. The name comes from the Ancient Greek word πλαγκτός (planktós), meaning "wandering" or "drifting", and bacterium, a Latin term coined in the 19th century by Christian Gottfried Ehrenberg. They are found in both seawater and fresh water.
Bacterioplankton occupy a range of ecological niches in marine and aquatic ecosystems. They are both primary producers and primary consumers in these ecosystems and drive global biogeochemical cycling of elements essential for life (e.g., carbon and nitrogen). Many bacterioplankton species are autotrophic, and derive energy from either photosynthesis or chemosynthesis. Photosynthetic bacterioplankton are often categorized as picophytoplankton, and include major cyanobacterial groups such as Prochlorococcus and Synechococcus. Other heterotrophic bacterioplankton are saprotrophic, and obtain energy by consuming organic material produced by other organisms. This material may be dissolved in the medium and taken directly from there, or bacteria may live and grow in association with particulate material such as marine snow. Bacterioplankton play critical roles in global nitrogen fixation, nitrification, denitrification, remineralisation and methanogenesis.
Bacterioplankton abundance depends on environmental variables like temperature, nutrient availability and predation. Like other small plankton, the bacterioplankton are preyed upon by zooplankton (usually protozoans), and their numbers are also controlled through infection by bacteriophages.
Major groups
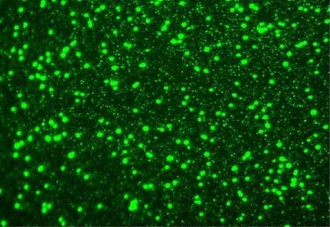
Photosynthetic bacterioplankton
Photosynthetic bacterioplankton are responsible for a large proportion of the total primary production of aquatic food webs, supplying organic compounds to higher trophic levels. These bacteria undergo oxygenic and anoxygenic photosynthesis. Differences between these processes can be seen in the byproducts produced, the primary electron donor, and the light harvesting pigments used for energy capture.

Cyanobacteria are a large group of photosynthetic bacterioplankton, often growing as cells or in filamentous colonies. These organisms are the dominant group of bacterioplankton using oxygenic photosynthesis in aquatic ecosystems. Cyanobacteria, along with photosynthetic eukaryotes, are responsible for approximately half of the total global primary production making them key players in the food web. They use photosynthesis to generate energy in the form of organic compounds and produce oxygen as a byproduct. Major light harvesting pigments include chlorophylls, phycoerytherin, phycocyanin and carotenoids. The majority of cyanobacteria found in marine environments are represented by the genera Synechococcus and Prochlorococcus. Synechococcus is cosmopolitan, having been reported across temperate and tropical waters. Prochlorococcus is a very small in size and is found mainly in the euphotic zone of tropical waters. Factors including light, nutrients, and temperature can cause cyanobacteria to proliferate and form harmful blooms. Cyanobacteria blooms can cause hypoxia and produce high levels of toxins, impacting other aquatic organisms as well as causing illnesses in humans.
Some Cyanobacteria are capable of nitrogen fixation. The genus Anabaena uses specialized cells called heterocysts to physically separate nitrogen fixation and photosynthesis. Trichodesmium is an example of cyanobacteria that is capable of fixing nitrogen through an alternative photosynthetic pathway.
Other photosynthetic bacterioplankton, including purple and green bacteria, undergo anoxygenic photosynthesis in anaerobic conditions. The pigments synthesized in these organisms are sensitive to oxygen. In purple bacteria the major pigments include bacteriochlorophyll a and b and carotenoids. Green bacteria have different light harvesting pigments consisting of bacteriochlorophyll c, d and e. These organisms do not produce oxygen through photosynthesis or use water as a reducing agent. Many of these organisms use sulfur, hydrogen or other compounds as an energy source to drive photosynthesis. Most of these bacterioplankton are found in anoxic waters, including stagnant and hypersaline environments.
Heterotrophic bacterioplankton
Heterotrophic bacterioplankton rely on the available concentration of dissolved organic matter in the water column. Usually these organisms are saprophytic, absorbing nutrients from their surroundings. These heterotrophs also play a key role in the microbial loop and the remineralization of organic compounds like carbon and nitrogen. Pelagibacterales (synonym SAR11), also known as members of an Alphaproteobacteria clade, are the most abundant bacterioplankton in the oceans. Members of this group are found in waters with low nutrient availability and are preyed on by protists.
Roseobacter is a diverse and widely-distributed clade which makes up a significant contribution of marine bacterioplankton, accounting up to roughly 20% of coastal waters and 15% mixed layer surface oceans. Although many are heterotrophic, some are capable of performing a unique form of photosynthesis called aerobic anoxygenic phototrophy, which requires rather than produces oxygen.
Biogeochemical cycling
Carbon
Atmospheric carbon is sequestered into the ocean by three main pumps which have been known for 30 years: the solubility pump, the carbonate pump, and the biological carbon pump (BCP). The biological carbon pump is a vertical transmission pump driven mainly by the sinking of organic rich particles. Bacterial phytoplankton near the surface incorporate atmospheric CO2 and other nutrients into their biomass during photosynthesis. At the time of their death these phytoplankton, along with their incorporated carbon, sink to the bottom of the ocean where the carbon remains for thousands of years. The other biologically mediated sequestration of carbon in the ocean occurs through the microbial pump. The microbial pump is responsible for the production of old recalcitrant dissolved organic carbon (DOC) which is >100 years old. Plankton in the ocean are incapable of breaking down this recalcitrant DOC and thus it remains in the oceans for 1000s years without being respired. The two pumps work simultaneously, and the balance between them is believed to vary based on the availability of nutrients. Overall, the oceans act as a sink for atmospheric CO2 but also release some carbon back into the atmosphere. This occurs when bacterioplankton and other organisms in the ocean consume organic matter and respire CO2, and as a result of the solubility equilibrium between the ocean and the atmosphere.
Nitrogen

The nitrogen cycle in the oceans is mediated by microorganisms, many of which are bacteria, performing multiple conversions such as: nitrogen fixation, denitrification, assimilation, and anaerobic ammonia oxidation (anammox). There are many different nitrogen metabolism strategies employed by bacterioplankton. Starting with molecular nitrogen in the atmosphere (N2), which is fixed by diazotrophs such as trichodesmium into usable forms like ammonia (NH
4). This ammonia can then be assimilated into organic matter like amino and nucleic acids, by both photoautrophic and heterotrophic plankton, it can also be nitrified to NO
3 for energy production by nitrifying bacteria. Finally the use of NO
3 or NO
2 as terminal electron acceptors reduces the nitrogen back into N2, which is then released back into the atmosphere thus closing the cycle. Another important process involved in the regeneration of atmospheric N2 is anammox. Anammox, a process in which ammonia is combined with nitrite in order to produce diatomic nitrogen and water, could account for 30–50% of production of N2 in the ocean.
An analysis on metagenomes of 83 species of cyanobacteria has suggested the possible dissimilatory nitrate reduction to ammonium (DNRA) activity in certain cyanobacteria. Namely, the study found the presence of the NirBD gene, which is a marker for DNRA function, in the families Leptolyngbyaceae and Nostocaceae. Moreover, the study indicated that the cyanobacteria that had NirBD are largely also non-heterocyst nitrogen fixers, suggesting possible alternative strategies of acquiring nitrogen under varying environmental conditions. Nonetheless, the NirBD gene is also known to play a role in nitrogen assimilation and further studies are required to ascertain the function of NirBD in cyanobacteria.
Dissolved organic matter
Dissolved organic matter (DOM) is available in many forms in the ocean, and is responsible for supporting the growth of bacteria and microorganisms in the ocean. The two main sources of this dissolved organic matter are; decomposition of higher trophic level organisms like plants and fish, and secondly DOM in runoffs that pass through soil with high levels of organic material. It is important to note that the age and quality of the DOM is important for its usability by microbes. The majority of the DOM in the oceans is refractory or semi-labile and is not available for biodegradation. As mentioned above the microbial pump is responsible for the production of refractory DOM which is unavailable for biodegradation and remains dissolved in the oceans for thousands of years. The turnover of labile DOM organic material is quite high due to scarcity, this is important for the support of multiple trophic levels in the microbial community. The uptake and respiration of DOM by heterotrophs closes the cycle by producing CO2.
Sulfur
Bacterioplankton, such as members of Roseobacter, SAR11, and Gammaproteobacteria, are known to contribute significantly towards the sulfur cycle, primarily through the metabolism of dimethylsulfoniopropionate (DMSP). DMSP can be catabolized either via means of cleavage to dimethylsulfide (DMS) or demethylation by bacterioplankton, in which both have contrasting effects on the sulfur cycle. The formation of DMS contributes to the sulfur flux into the atmosphere and according to the CLAW hypothesis, plays a role in regulating global climate. Increased production of sulfate aerosols from DMS oxidation are capable of promoting cooling on a global scale, via the promotion of cloud formation. In contrast, the demethylation pathway from DMSP to methanethiol results in the integration of carbon and sulfur into the organism itself as opposed to releasing the elements back to the environment. Bacterioplankton DMSP degradation is thought to be prevalent in marine surface waters, although the spatial distribution of the two aforementioned routes of degradation exhibit high variation.
Similar to DNRA, the same study indicated the presence of a dsyB-like gene in certain cyanobacteria genomes, suggesting DMSP producing ability. However, there has yet to be empirical confirmation of DMSP synthesis in cyanobacteria.
Silica
Diatoms are a major group of phytoplankton in which most have a requirement for silicon as biogenic silica to form their cell wall (known as frustule). Upon predation or death, particulate silica is released from diatoms but they need to be dissolved for recycling and reuptake by diatoms, otherwise silica will be exported out and deposited into sediment. Hence, the productivity of diatoms will be limited by silicon if dissolution rates are slow. However, it is known that bacterioplankton (i.e. members of Cytophaga-Flavobacterium-Bacteroides, Alphaproteobacteria, and Gammaproteobacteria) significantly promote the dissolution of particulate silica, thus maintaining the significant biogenic silica production in the ocean photic zone. It is also suggested that this process helps regulate diatom productivity and its corresponding biogeochemical effects.
Trophic interactions

Variations in bacterioplankton abundance are usually a result of temperature, zooplankton grazing, and availability of substrate. Bacterial abundance and productivity are consistently related to algal abundance and productivity as well as organic carbon. Additionally, phosphorus directly influences both algal and bacterial abundance and in turn, algae and bacteria directly influence each other's abundance In extremely oligotrophic environments, both bacterial and algal growth is limited by phosphorus, but because bacteria are better competitors they obtain a larger portion of the inorganic substrate and increase in abundance more rapidly than algae.
In marine pelagic environments, heterotrophic nano-flagellates are the most probable consumers of bacterial cell production. Cultured flagellates in laboratory experiments demonstrate that they are adapted to predation on bacteria-sized particles and occur in concentrations to control bacterial biomass. Tight fluctuations in numbers of bacteria and flagellates have been found in a eutrophic estuary, particularly in the summer. The amplitude of these fluctuations increases in response to artificial eutrophication with inorganic nutrients and decreases in response to predation. Losses of bacterioplankton by grazing is indirectly related to carbon balances and directly related to prokaryotic inhibitors. A surplus of substrate would cause increased flagellate biomass, increased grazing on bacterioplankton and therefore decreased bacterial biomass overall. Predation of ciliates is analogous to predation by flagellates on bacteria as well.
With using prokaryotic inhibitors seasonally, there is a positive relationship between bacterial abundance and heterotrophic nanoplankton grazing rates and only 40-45 % of bacterioplankton production was observed to be consumed by phagotrophic Protozoa. Additionally, eukaryotic inhibitory experiments show that protozoan grazing has a positive effect on bacterioplankton production suggesting that nitrogen regeneration by Protozoa could be highly important for bacterial growth. Eukaryotic inhibitors did not prove to be useful to determine protozoan grazing rates on bacterioplankton, however they may help understand control mechanisms in the microbial food web.
Ecological significance

Bacterioplankton such as cyanobacteria are able to have toxic blooms in eutrophic lakes which can lead to the death of many organisms such as fish, birds, cattle, pets and humans. A few examples of these harmful blooms is the Microcystis bloom in the year 2000 in Swan River estuary, Australia, and the Oostvaarderplassen in the Netherlands in 2003. The detrimental effects of these blooms can range from heart malformation in fish to constraining copepod reproduction.
High temperatures caused by seasonality increases stratification and preventing vertical turbulent mixing which increases competition for light that favours buoyant cyanobacteria. Higher temperatures also reduce the viscosity of water which allows faster movement which also favors buoyant species of cyanobacteria. These species are also very competitive with the ability to create a surface cover preventing light to reach deeper species of plankton.
Climate studies are also indicating that with increasing hot waves the likelihood of detrimental cyanobacterial blooms will become more of a threat to eutrophic freshwater systems. Other implications of the increasing average air temperature due to climate change is that there might be an expansion of the cyanobacterial bloom season, extending from earlier in the spring to later in the fall.
Estimates of bacterioplankton abundance and density can be derived with a variety of methods including direct counts, flow cytometry, and conclusions drawn from metabolic measures.
Further, as discussed in the biogeochemical cycling section, plankton are responsible for the recycling and movement of essential nutrients (i.e. nitrogen/carbon/DOM) which are essential building blocks for many of the organisms co-existing with bacterioplankton in these ecosystems. These recycled nutrients can be reused by primary producers, thus increasing the efficiency of the biological food web and minimizing energy waste.
See also
- Bacterioplankton counting methods
- Cyanobacteria
- Pelagibacter
- Polynucleobacter
- Limnohabitans
- Roseobacter
- Phytoplankton
- Plankton
- Zooplankton
- Marine bacteria
- Marine bacteriophage
References
- ^ Mann NH, Carr NG, eds. (1992). Photosynthetic Prokaryotes. doi:10.1007/978-1-4757-1332-9. ISBN 978-1-4757-1334-3. S2CID 6924271.
- Field CB, Behrenfeld MJ, Randerson JT, Falkowski P (July 1998). "Primary production of the biosphere: integrating terrestrial and oceanic components". Science. 281 (5374): 237–240. Bibcode:1998Sci...281..237F. doi:10.1126/science.281.5374.237. PMID 9657713. S2CID 45140824.
- Peschek GA, Bernroitner M, Sari S, Pairer M, Obinger C (2011). Bioenergetic Processes of Cyanobacteria. Springer, Dordrecht. pp. 3–70. doi:10.1007/978-94-007-0388-9_1. ISBN 9789400703520.
- Colyer CL, Kinkade CS, Viskari PJ, Landers JP (June 2005). "Analysis of cyanobacterial pigments and proteins by electrophoretic and chromatographic methods". Analytical and Bioanalytical Chemistry. 382 (3): 559–569. doi:10.1007/s00216-004-3020-4. PMID 15714301. S2CID 35388030.
- Johnson PW, Sieburth JM (September 1979). "Chroococcoid cyanobacteria in the sea: A ubiquitous and diverse phototrophic biomass1". Limnology and Oceanography. 24 (5): 928–935. Bibcode:1979LimOc..24..928J. doi:10.4319/lo.1979.24.5.0928. ISSN 1939-5590.
- Chisholm SW, Frankel SL, Goericke R, Olson RJ, Palenik B, Waterbury JB, et al. (1992-02-01). "Prochlorococcus marinus nov. gen. nov. sp.: an oxyphototrophic marine prokaryote containing divinyl chlorophyll a and b". Archives of Microbiology. 157 (3): 297–300. Bibcode:1992ArMic.157..297C. doi:10.1007/bf00245165. ISSN 0302-8933. S2CID 32682912.
- Chisholm SW, Olson RJ, Zettler ER, Goericke R, Waterbury JB, Welschmeyer NA (July 1988). "A novel free-living prochlorophyte abundant in the oceanic euphotic zone". Nature. 334 (6180): 340–343. Bibcode:1988Natur.334..340C. doi:10.1038/334340a0. ISSN 1476-4687. S2CID 4373102.
- Reynolds CS, Walsby AE (1975-11-01). "Water-Blooms". Biological Reviews. 50 (4): 437–481. doi:10.1111/j.1469-185x.1975.tb01060.x. ISSN 1469-185X. S2CID 221529895.
- Agnihotri VK (2014). "Anabaena flos-aquae". Critical Reviews in Environmental Science and Technology. 44 (18): 1995–2037. Bibcode:2014CREST..44.1995A. doi:10.1080/10643389.2013.803797. S2CID 84472933.
- Bergman B, Sandh G, Lin S, Larsson J, Carpenter EJ (May 2013). "Trichodesmium--a widespread marine cyanobacterium with unusual nitrogen fixation properties". FEMS Microbiology Reviews. 37 (3): 286–302. doi:10.1111/j.1574-6976.2012.00352.x. PMC 3655545. PMID 22928644.
- Kopylov AI, Kosolapov DB, Degermendzhy NN, Zotina TA, Romanenko AV (April 2002). "Phytoplankton, bacterial production and protozoan bacterivory in stratified, brackish-water Lake Shira (Khakasia, Siberia)". Aquatic Ecology. 36 (2): 205–218. doi:10.1023/a:1015611023296. ISSN 1386-2588. S2CID 20520535.
- Morris RM, Rappé MS, Connon SA, Vergin KL, Siebold WA, Carlson CA, et al. (December 2002). "SAR11 clade dominates ocean surface bacterioplankton communities". Nature. 420 (6917): 806–810. Bibcode:2002Natur.420..806M. doi:10.1038/nature01240. PMID 12490947. S2CID 4360530.
- Cole JJ, Findlay S, Pace ML (1988). "Bacterial production in fresh and saltwater ecosystems: a cross-system overview". Marine Ecology Progress Series. 43: 1–10. Bibcode:1988MEPS...43....1C. doi:10.3354/meps043001.
- Luo H, Moran MA (December 2014). "Evolutionary ecology of the marine Roseobacter clade". Microbiology and Molecular Biology Reviews. 78 (4): 573–587. doi:10.1128/MMBR.00020-14. PMC 4248658. PMID 25428935.
- ^ Buchan A, González JM, Moran MA (October 2005). "Overview of the marine roseobacter lineage". Applied and Environmental Microbiology. 71 (10): 5665–5677. Bibcode:2005ApEnM..71.5665B. doi:10.1128/AEM.71.10.5665-5677.2005. PMC 1265941. PMID 16204474.
- ^ Legendre L, Rivkin RB, Weinbauer MG, Guidi L, Uitz J (2015-05-01). "The microbial carbon pump concept: Potential biogeochemical significance in the globally changing ocean". Progress in Oceanography. 134: 432–450. Bibcode:2015PrOce.134..432L. doi:10.1016/j.pocean.2015.01.008. ISSN 0079-6611.
- De La Rocha CL, Passow U (2014). Treatise on Geochemistry. pp. 93–122. doi:10.1016/b978-0-08-095975-7.00604-5. ISBN 9780080983004.
- Polimene L, Sailley S, Clark D, Mitra A, Allen JI (2017-03-01). "Biological or microbial carbon pump? The role of phytoplankton stoichiometry in ocean carbon sequestration". Journal of Plankton Research. 39 (2). doi:10.1093/plankt/fbw091. ISSN 0142-7873.
- Follows M, Oguz T, et al. (North Atlantic Treaty Organization. Scientific Affairs Division.) (2004). The ocean carbon cycle and climate. Dordrecht: Kluwer Academic Publishers. ISBN 9781402020872. OCLC 54974524.
- Bergman B, Sandh G, Lin S, Larsson J, Carpenter EJ (May 2013). "Trichodesmium--a widespread marine cyanobacterium with unusual nitrogen fixation properties". FEMS Microbiology Reviews. 37 (3): 286–302. doi:10.1111/j.1574-6976.2012.00352.x. PMC 3655545. PMID 22928644.
- ^ Zehr JP, Kudela RM (2011). "Nitrogen cycle of the open ocean: from genes to ecosystems". Annual Review of Marine Science. 3 (1): 197–225. Bibcode:2011ARMS....3..197Z. doi:10.1146/annurev-marine-120709-142819. PMID 21329204.
- ^ Reimann J, Jetten MS, Keltjens JT (2015). "Metal Enzymes in "Impossible" Microorganisms Catalyzing the Anaerobic Oxidation of Ammonium and Methane". Sustaining Life on Planet Earth: Metalloenzymes Mastering Dioxygen and Other Chewy Gases. Metal Ions in Life Sciences. Vol. 15. Springer, Cham. pp. 257–313. doi:10.1007/978-3-319-12415-5_7. ISBN 9783319124148. PMID 25707470.
- ^ Ray M, Manu S, Rastogi G, Umapathy G (April 2024). "Cyanobacterial Genomes from a Brackish Coastal Lagoon Reveal Potential for Novel Biogeochemical Functions and Their Evolution". Journal of Molecular Evolution. 92 (2): 121–137. Bibcode:2024JMolE..92..121R. doi:10.1007/s00239-024-10159-y. PMID 38489069.
- Malm S, Tiffert Y, Micklinghoff J, Schultze S, Joost I, Weber I, et al. (April 2009). "The roles of the nitrate reductase NarGHJI, the nitrite reductase NirBD and the response regulator GlnR in nitrate assimilation of Mycobacterium tuberculosis". Microbiology. 155 (Pt 4): 1332–1339. doi:10.1099/mic.0.023275-0. PMID 19332834.
- Søndergaard M, Middelboe M (1995-03-09). "A cross-system analysis of labile dissolved organic carbon". Marine Ecology Progress Series. 118: 283–294. Bibcode:1995MEPS..118..283S. doi:10.3354/meps118283. ISSN 0171-8630.
- Gruber DF, Simjouw JP, Seitzinger SP, Taghon GL (June 2006). "Dynamics and characterization of refractory dissolved organic matter produced by a pure bacterial culture in an experimental predator-prey system". Applied and Environmental Microbiology. 72 (6): 4184–4191. Bibcode:2006ApEnM..72.4184G. doi:10.1128/aem.02882-05. PMC 1489638. PMID 16751530.
- Kirchman DL, Suzuki Y, Garside C, Ducklow HW (1991). "High turnover rates of dissolved organic carbon during a spring phytoplankton bloom". Nature. 352 (6336): 612–614. Bibcode:1991Natur.352..612K. doi:10.1038/352612a0. ISSN 1476-4687. S2CID 4285758.
- Howard EC, Sun S, Biers EJ, Moran MA (September 2008). "Abundant and diverse bacteria involved in DMSP degradation in marine surface waters". Environmental Microbiology. 10 (9): 2397–2410. Bibcode:2008EnvMi..10.2397H. doi:10.1111/j.1462-2920.2008.01665.x. PMID 18510552.
- Reisch CR, Moran MA, Whitman WB (2011). "Bacterial Catabolism of Dimethylsulfoniopropionate (DMSP)". Frontiers in Microbiology. 2: 172. doi:10.3389/fmicb.2011.00172. PMC 3155054. PMID 21886640.
- González JM, Kiene RP, Moran MA (September 1999). "Transformation of sulfur compounds by an abundant lineage of marine bacteria in the alpha-subclass of the class Proteobacteria". Applied and Environmental Microbiology. 65 (9): 3810–3819. Bibcode:1999ApEnM..65.3810G. doi:10.1128/AEM.65.9.3810-3819.1999. PMC 99705. PMID 10473380.
- ^ Charlson RJ, Lovelock JE, Andreae MO, Warren SG (April 1987). "Oceanic phytoplankton, atmospheric sulphur, cloud albedo and climate". Nature. 326 (6114): 655–661. Bibcode:1987Natur.326..655C. doi:10.1038/326655a0. ISSN 0028-0836.
- Yoch DC (December 2002). "Dimethylsulfoniopropionate: its sources, role in the marine food web, and biological degradation to dimethylsulfide". Applied and Environmental Microbiology. 68 (12): 5804–5815. Bibcode:2002ApEnM..68.5804Y. doi:10.1128/AEM.68.12.5804-5815.2002. PMC 134419. PMID 12450799.
- Moran MA, González JM, Kiene RP (July 2003). "Linking a Bacterial Taxon to Sulfur Cycling in the Sea: Studies of the Marine Roseobacter Group". Geomicrobiology Journal. 20 (4): 375–388. Bibcode:2003GmbJ...20..375M. doi:10.1080/01490450303901. ISSN 0149-0451.
- Cui Y, Suzuki S, Omori Y, Wong SK, Ijichi M, Kaneko R, et al. (June 2015). Drake HL (ed.). "Abundance and distribution of dimethylsulfoniopropionate degradation genes and the corresponding bacterial community structure at dimethyl sulfide hot spots in the tropical and subtropical pacific ocean". Applied and Environmental Microbiology. 81 (12): 4184–4194. Bibcode:2015ApEnM..81.4184C. doi:10.1128/AEM.03873-14. PMC 4524131. PMID 25862229.
- Bidle KD, Azam F (February 1999). "Accelerated dissolution of diatom silica by marine bacterial assemblages". Nature. 397 (6719): 508–512. Bibcode:1999Natur.397..508B. doi:10.1038/17351. ISSN 0028-0836.
- Bidle KD, Brzezinski MA, Long RA, Jones JL, Azam F (September 2003). "Diminished efficiency in the oceanic silica pump caused by bacteria-mediated silica dissolution". Limnology and Oceanography. 48 (5): 1855–1868. Bibcode:2003LimOc..48.1855B. doi:10.4319/lo.2003.48.5.1855. ISSN 0024-3590.
- ^ Currie DJ (1990-11-01). "Large-scale variability and interactions among phytoplankton, bacterioplankton, and phosphorus". Limnology and Oceanography. 35 (7): 1437–1455. Bibcode:1990LimOc..35.1437C. doi:10.4319/lo.1990.35.7.1437. ISSN 1939-5590.
- ^ Fenchel T (1982). "Ecology of Heterotrophic Microflagellates. IV. Quantitative Occurrence and Importance as Bacterial Consumers". Marine Ecology Progress Series. 9 (1): 35–42. Bibcode:1982MEPS....9...35F. doi:10.3354/meps009035. JSTOR 24814568.
- Porter KG, Sherr EB, Sherr BF, Pace M, Sanders RW (August 1985). "Protozoa in Planktonic Food Webs1,2". The Journal of Protozoology. 32 (3): 409–415. doi:10.1111/j.1550-7408.1985.tb04036.x. ISSN 1550-7408.
- Andersen P, Sørensen HM (1986). "Population dynamics and trophic coupling in pelagic microorganisms in eutrophic coastal waters". Marine Ecology Progress Series. 33 (2): 99–109. Bibcode:1986MEPS...33...99A. doi:10.3354/meps033099. JSTOR 24821288.
- Bjørnsen PK, Riemann B, Horsted SJ, Nielsen TG, Pock-Sten J (May 1988). "Trophic interactions between heterotrophic nanoflagellates and bacterioplankton in manipulated seawater enclosures1". Limnology and Oceanography. 33 (3): 409–420. Bibcode:1988LimOc..33..409B. doi:10.4319/lo.1988.33.3.0409. ISSN 1939-5590.
- ^ Sherr BF, Sherr EB, Andrew TL, Fallon RD, Newell SY (1986). "Trophic interactions between heterotrophic Protozoa and bacterioplankton in estuarine water analyzed with selective metabolic inhibitors". Marine Ecology Progress Series. 32 (2/3): 169–179. Bibcode:1986MEPS...32..169S. doi:10.3354/meps032169. JSTOR 24824977.
- ^ Jöhnk KD, Huisman J, Sharples J, Sommeijer B, Visser PM, Stroom JM (2008). "Summer heatwaves promote blooms of harmful cyanobacteria". Global Change Biology. 14 (3): 495–512. Bibcode:2008GCBio..14..495J. doi:10.1111/j.1365-2486.2007.01510.x. S2CID 54079634.
- Atkins R, Rose T, Brown RS, Robb M (2001). "The Microcystis cyanobacteria bloom in the Swan River--February 2000". Water Science and Technology. 43 (9): 107–114. doi:10.2166/wst.2001.0518. PMID 11419118.
- Kardinaal WE, Visser PM. "Cyanotoxines Drijven tot Overlast: Inventarisatie van Microcystine Concentraties 2000–2004 in Nederlandse Oppervlakte Wateren". Report for the National Institute for Inland Water Management and Wastewater Treatment, the Netherlands: 23 pp.
- Zi J, Pan X, MacIsaac HJ, Yang J, Xu R, Chen S, et al. (January 2018). "Cyanobacteria blooms induce embryonic heart failure in an endangered fish species". Aquatic Toxicology. 194: 78–85. Bibcode:2018AqTox.194...78Z. doi:10.1016/j.aquatox.2017.11.007. PMID 29169051.
- Engström-Öst J, Brutemark A, Vehmaa A, Motwani NH, Katajisto T (2015). "Consequences of a cyanobacteria bloom for copepod reproduction, mortality and sex ratio". Journal of Plankton Research. 37 (2): 388–398. doi:10.1093/plankt/fbv004.
- ^ Walsby AE, Hayes PK, Boje R, Stal LJ (July 1997). "The selective advantage of buoyancy provided by gas vesicles for planktonic cyanobacteria in the Baltic Sea". The New Phytologist. 136 (3): 407–417. doi:10.1046/j.1469-8137.1997.00754.x. PMID 33863010.
- ^ Huisman J, Sharples J, Stroom J, Visser PM, Kardinaal WE, Verspagen JM, et al. (2004). "Changes in turbulent mixing shift competition for light between phytoplankton species". Ecology. 85 (11): 2960–2970. Bibcode:2004Ecol...85.2960H. doi:10.1890/03-0763.
- Klausmeier CA, Litchman E (2001). "Algal games: the vertical distribution of phytoplankton in poorly mixed water columns". Limnology and Oceanography. 46 (8): 1998–2007. Bibcode:2001LimOc..46.1998K. doi:10.4319/lo.2001.46.8.1998.
- Beniston M (2004). "The 2003 heat wave in Europe: a shape of things to come? An analysis based on Swiss climatological data and model simulations" (PDF). Geophysical Research Letters. 31 (2): L02202. Bibcode:2004GeoRL..31.2202B. doi:10.1029/2003gl018857.
- Schär C, Vidale PL, Lüthi D, Frei C, Häberli C, Liniger MA, et al. (January 2004). "The role of increasing temperature variability in European summer heatwaves". Nature. 427 (6972): 332–336. Bibcode:2004Natur.427..332S. doi:10.1038/nature02300. PMID 14716318. S2CID 4431020.
- Stott PA, Stone DA, Allen MR (December 2004). "Human contribution to the European heatwave of 2003". Nature. 432 (7017): 610–614. Bibcode:2004Natur.432..610S. doi:10.1038/nature03089. PMID 15577907. S2CID 13882658.
- Srifa A, Phlips EJ, Cichra MF, Hendrickson JC (2016). "Phytoplankton dynamics in a subtropical lake dominated by cyanobacteria: Cyanobacteria 'Like it hot' and sometimes dry". Aquatic Ecology. 50 (2): 163–174. Bibcode:2016AqEco..50..163S. doi:10.1007/s10452-016-9565-4. S2CID 10528364.
Further reading
- Thurman HV (1997). Introductory Oceanography. New Jersey, USA: Prentice Hall College. ISBN 978-0-13-262072-7.