
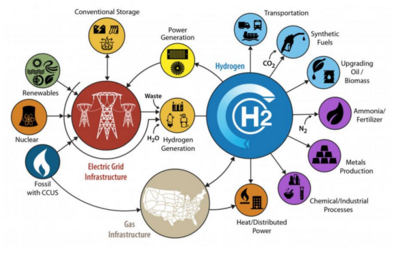
High-temperature electrolysis (also HTE or steam electrolysis, or HTSE) is a technology for producing hydrogen from water at high temperatures or other products, such as iron or carbon nanomaterials, as higher energy lowers needed electricity to split molecules and opens up new, potentially better electrolytes like molten salts or hydroxides. Unlike electrolysis at room temperature, HTE operates at elevated temperature ranges depending on the thermal capacity of the material. Because of the detrimental effects of burning fossil fuels on humans and the environment, HTE has become a necessary alternative and efficient method by which hydrogen can be prepared on a large scale and used as fuel. The vision of HTE is to move towards decarbonization in all economic sectors. The material requirements for this process are: the heat source, the electrodes, the electrolyte, the electrolyzer membrane, and the source of electricity.
Principle
The process utilizes energy (in the form of heat) from sources to convert water into steam, which is then passed into an electrolytic system (made up of two electrodes connected to the source of current, an electrolyte, and a membrane). At high temperatures (over 650 °C in most topologies), the materials used to construct the cells become conductive. Therefore, electrochemical reactions begin to occur, and the cell begins to function once it has reached the proper temperature and electricity is supplied while it is being fed with steam. The steam will eventually split into hydrogen (cathode) and oxygen (anode) according to the equations below:
Efficiency

60% efficient at 1000°C
Steam reforming of hydrocarbons to hydrogen is 70-85% efficient
High temperature electrolysis is more efficient economically than traditional room-temperature electrolysis because some of the energy is supplied as heat, which is cheaper than electricity, and also because the electrolysis reaction is more efficient at higher temperatures. In fact, at 2500 °C, electrical input is unnecessary because water breaks down to hydrogen and oxygen through thermolysis. Such temperatures are impractical; proposed HTE systems operate between 100 °C and 850 °C.
If one assumes that the electricity used comes from a heat engine, it takes 141.86 megajoules (MJ) of heat energy to produce one kg of hydrogen, for the HTE process itself and for the electricity required. At 100 °C, 350 MJ of thermal energy are required (41% efficient). At 850 °C, 225 MJ are required (64% efficient). Above 850 °C, one begins to exceed the capacity of standard chromium steels to resist corrosion, and it's already no easy matter to design and implement an industrial scale chemical process to operate at such a high temperature point.
Materials
Solid oxide electrolysis cells (SOECs) are electrochemical devices that function at high temperatures and are used for high-temperature electrolysis. These cells' ingredients ensure that the device will function well both physically and electrochemically at high temperatures. Therefore, the selection of materials for the electrodes and electrolyte in a solid oxide electrolyser cell is essential. One option being investigated for the process used yttria-stabilized zirconia (YSZ) electrolytes, Nickel (Ni)-cermet steam/Hydrogen electrodes, and d Oxide of Lanthanum oxide (La2O3), Strontium and Cobalt oxygen electrodes.
Economic potential
Even with HTE, electrolysis is a fairly inefficient way to store energy. Significant conversion losses of energy occur both in the electrolysis process, and in the conversion of the resulting hydrogen back into power.
At current hydrocarbon prices, HTE can not compete with pyrolysis of hydrocarbons as an economical source of hydrogen, which produces carbon dioxide as a by-product.
HTE is of interest as a more efficient route to the production "green" hydrogen, to be used as a carbon neutral fuel and general energy storage. It may become economical if cheap non-fossil fuel sources of heat (concentrating solar, nuclear, geothermal, waste heat) can be used in conjunction with non-fossil fuel sources of electricity (such as solar, wind, ocean, nuclear).
Possible supplies of cheap high-temperature heat for HTE are all nonchemical, including nuclear reactors, concentrating solar thermal collectors, and geothermal sources. HTE has been demonstrated in a laboratory at 108 kilojoules (electric) per gram of hydrogen produced, but not at a commercial scale.
Advantages and Challenges
Obviously, the most notable advantage of HTE is that it provides an opportunity for which green hydrogen is prepared on a large scale, because it has the potential for zero emissions. The process provides an improved reaction kinetics for the splitting of water molecule. Part of the electricity requirement is replaced with heat, which makes it a bit cheaper because electricity is more expensive than heat.
However, HTE technology suffered limitations due to:
- Above 100 °C, the electrolysis of liquid water requires pressurization, and is therefore limited by the working pressures that can be reasonably attained.
- creating materials that are both chemically and physically stable in conditions of intense oxidation and reduction, as well as high working temperatures.
- chemical and physical stability at low electrical conductivities, high working temperatures, and/or ionic concentrations.
Alternatives
There are hundreds of thermochemical cycles known to use heat to extract hydrogen from water. For instance, the thermochemical sulfur-iodine cycle. Since the electricity generation step has a fairly low efficiency and is eliminated, thermochemical production might reach higher efficiencies than HTE. However, large-scale thermochemical production will require significant advances in materials that can withstand high-temperature, high-pressure, highly corrosive environments.
United States Department of Energy
See also: Next Generation Nuclear PlantThe DOE Office of Nuclear Energy has demonstration projects to test 3 nuclear facilities with high-temperature electrolysis in the United States at:
- Nine Mile Point Nuclear Generating Station in Oswego, NY
- Davis–Besse Nuclear Power Station in Oak Harbor, Ohio
- Prairie Island Nuclear Power Plant in Red Wing, Minnesota
Mars ISRU
See also: In-situ resource utilizationHigh temperature electrolysis with solid oxide electrolyser cells was used to produce 5.37 grams of oxygen per hour on Mars from atmospheric carbon dioxide for the Mars Oxygen ISRU Experiment in the NASA Mars 2020 Perseverance rover, using zirconia electrolysis devices.
See also
References
Footnotes
- Hauch, A.; Ebbesen, S. D.; Jensen, S. H.; Mogensen, M. (2008). "Highly Efficient high temperature electrolysis". J. Mater. Chem. 18 (20): 2331–2340. doi:10.1039/b718822f.
- Licht, Stuart; Wu, Hongjun (2011-12-22). "STEP Iron, a Chemistry of Iron Formation without CO 2 Emission: Molten Carbonate Solubility and Electrochemistry of Iron Ore Impurities". The Journal of Physical Chemistry C. 115 (50): 25138–25147. doi:10.1021/jp2078715. ISSN 1932-7447.
- Licht, Stuart; Cui, Baochen; Wang, Baohui (2013-09-01). "STEP carbon capture – The barium advantage". Journal of CO2 Utilization. 2: 58–63. Bibcode:2013JCOU....2...58L. doi:10.1016/j.jcou.2013.03.006. ISSN 2212-9820.
- Ren, Jiawen; Yu, Ao; Peng, Ping; Lefler, Matthew; Li, Fang-Fang; Licht, Stuart (2019-11-19). "Recent Advances in Solar Thermal Electrochemical Process (STEP) for Carbon Neutral Products and High Value Nanocarbons". Accounts of Chemical Research. 52 (11): 3177–3187. doi:10.1021/acs.accounts.9b00405. ISSN 0001-4842. PMID 31697061.
- Valderrama, César (2016), "High-Temperature Electrolysis", in Drioli, Enrico; Giorno, Lidietta (eds.), Encyclopedia of Membranes, Berlin, Heidelberg: Springer, pp. 937–939, doi:10.1007/978-3-662-44324-8_2122, ISBN 978-3-662-44324-8, retrieved 2024-04-14
- "Fact Sheet | Climate, Environmental, and Health Impacts of Fossil Fuels (2021) | White Papers". www.eesi.org. Retrieved 2024-04-14.
- Shiva Kumar, S.; Lim, Hankwon (November 2022). "An overview of water electrolysis technologies for green hydrogen production". Energy Reports. 8: 13793–13813. Bibcode:2022EnRep...813793S. doi:10.1016/j.egyr.2022.10.127. ISSN 2352-4847.
- ^ Zainal, Bidattul Syirat; Ker, Pin Jern; Mohamed, Hassan; Ong, Hwai Chyuan; Fattah, I.M.R.; Rahman, S.M. Ashrafur; Nghiem, Long D.; Mahlia, T M Indra (January 2024). "Recent advancement and assessment of green hydrogen production technologies". Renewable and Sustainable Energy Reviews. 189: 113941. Bibcode:2024RSERv.18913941Z. doi:10.1016/j.rser.2023.113941. ISSN 1364-0321.
- ^ Crema, Luigi; Testi, Matteo; Trini, Martina (2021-09-07), 5 High-temperature electrolysis: efficient and versatile solution for multiple applications, De Gruyter, pp. 219–268, doi:10.1515/9783110596274-013, ISBN 978-3-11-059627-4, retrieved 2024-04-14
- Mougin, J. (2015), "Hydrogen production by high-temperature steam electrolysis", Compendium of Hydrogen Energy, Elsevier, pp. 225–253, doi:10.1016/b978-1-78242-361-4.00008-x, ISBN 978-1-78242-361-4, retrieved 2024-04-14
- https://inldigitallibrary.inl.gov/sites/sti/sti/4480292.pdf
- Kalamaras, Christos M.; Efstathiou, Angelos M. (2013). "Hydrogen Production Technologies: Current State and Future Developments". Conference Papers in Energy. 2013: 1–9. doi:10.1155/2013/690627.
- Badwal, SPS; Giddey S; Munnings C (2012). "Hydrogen production via solid electrolytic routes". WIREs Energy and Environment. 2 (5): 473–487. doi:10.1002/wene.50. S2CID 135539661.
- Hi2h2 - High temperature electrolysis using SOEC
- Final Report Summary – WELTEMP (Water Electrolysis at Elevated Temperatures)
- "Stainless Steel - High Temperature Resistance". azom.com. AZO Materials. 8 January 2002. Retrieved 6 August 2021.
Most austenitic steels, with chromium contents of at least 18%, can be used at temperatures up to 870°C and even higher.
- Kazuya Yamada, Shinichi Makino, Kiyoshi Ono, Kentaro Matsunaga, Masato Yoshino, Takashi Ogawa, Shigeo Kasai, Seiji Fujiwara, and Hiroyuki Yamauchi "High Temperature Electrolysis for Hydrogen Production Using Solid Oxide Electrolyte Tubular Cells Assembly Unit", presented at AICHE Annual Meeting, San Francisco, California, November 2006. abstract
- "Steam heat: researchers gear up for full-scale hydrogen plant" (Press release). Science Daily. 2008-09-19.
- "Nuclear hydrogen R&D plan" (PDF). U.S. Dept. of Energy. March 2004. Archived from the original (PDF) on 2013-09-24. Retrieved 2008-05-09.
- Elder, Rachael; Cumming, Denis; Mogensen, Mogens Bjerg (2015), "High Temperature Electrolysis", Carbon Dioxide Utilisation, Elsevier, pp. 183–209, doi:10.1016/b978-0-444-62746-9.00011-6, ISBN 978-0-444-62746-9, retrieved 2024-04-14
- Lohmann-Richters, F. P.; Renz, S.; Lehnert, W.; Müller, M.; Carmo, M. (2021-11-01). "Review—Challenges and Opportunities for Increased Current Density in Alkaline Electrolysis by Increasing the Operating Temperature". Journal of the Electrochemical Society. 168 (11): 114501. Bibcode:2021JElS..168k4501L. doi:10.1149/1945-7111/ac34cc. ISSN 0013-4651.
- ^ Acar, Canan; Dincer, Ibrahim (2018-01-01), Dincer, Ibrahim (ed.), "3.1 Hydrogen Production", Comprehensive Energy Systems, Oxford: Elsevier, pp. 1–40, doi:10.1016/b978-0-12-809597-3.00304-7, ISBN 978-0-12-814925-6, retrieved 2024-04-14
- "3 Nuclear Power Plants Gearing up for Clean Hydrogen Production".
- Wall, Mike (August 1, 2014). "Oxygen-Generating Mars Rover to Bring Colonization Closer". Space.com. Retrieved 2014-11-05.
- The Mars Oxygen ISRU Experiment (MOXIE) PDF. Presentation: MARS 2020 Mission and Instruments". November 6, 2014.
- Potter, Sean (2021-04-21). "NASA's Perseverance Mars Rover Extracts First Oxygen from Red Planet". NASA. Retrieved 2021-04-22.
Articles related to electrolysis / Standard electrode potential | |
---|---|
Electrolytic processes | |
Materials produced by electrolysis | |
See also |