
In zoology, megafauna (from Greek μέγας megas "large" and Neo-Latin fauna "animal life") are large animals. The precise definition of the term varies widely, though a common threshold is approximately 45 kilograms (99 lb), with other thresholds as low as 10 kilograms (22 lb) or as high as 1,000 kilograms (2,200 lb). Large body size is generally associated with other traits, such as having a slow rate of reproduction and, in large herbivores, reduced or negligible adult mortality from being killed by predators.
Megafauna species have considerable effects on their local environment, including the suppression of the growth of woody vegetation and a consequent reduction in wildfire frequency. Megafauna also play a role in regulating and stabilizing the abundance of smaller animals.
During the Pleistocene, megafauna were diverse across the globe, with most continental ecosystems exhibiting similar or greater species richness in megafauna as compared to ecosystems in Africa today. During the Late Pleistocene, particularly from around 50,000 years ago onwards, most large mammal species became extinct, including 80% of all mammals greater than 1,000 kilograms (2,200 lb), while small animals were largely unaffected. This pronouncedly size-biased extinction is otherwise unprecedented in the geological record. Humans and climatic change have been implicated by most authors as the likely causes, though the relative importance of either factor has been the subject of significant controversy.
History
One of the earliest occurrences of the term "megafauna" is Alfred Russel Wallace's 1876 work The geographical distribution of animals. He described the animals as "the hugest, and fiercest, and strangest forms". In the 20th and 21st centuries, the term usually refers to large animals. There are variations in thresholds used to define megafauna as a whole or certain groups of megafauna. Many scientific literature adopt Paul S. Martin's proposed threshold of 45 kilograms (99 lb) to classify animals as megafauna. However, for freshwater species, 30 kilograms (66 lb) is the preferred threshold. Some scientists define herbivorous terrestrial megafauna as having a weight exceeding 100 kilograms (220 lb), and terrestrial carnivorous megafauna as more than 15 kilograms (33 lb). Additionally, Owen-Smith coined the term megaherbivore to describe herbivores that weighed over 1,000 kilograms (2,200 lb), which has seen some use by other researchers.
Among living animals, the term megafauna is most commonly used for the largest extant terrestrial mammals, which includes (but is not limited to) elephants, giraffes, hippopotamuses, rhinoceroses, and larger bovines. Of these five categories of large herbivores, only bovines are presently found outside of Africa and Asia, but all the others were formerly more wide-ranging, with their ranges and populations continually shrinking and decreasing over time. Wild equines are another example of megafauna, but their current ranges are largely restricted to the Old World, specifically in Africa and Asia. Megafaunal species may be categorized according to their dietary type: megaherbivores (e.g., elephants), megacarnivores (e.g., lions), and megaomnivores (e.g., bears).
Ecological strategy
Megafauna animals – in the sense of the largest mammals and birds – are generally K-strategists, with high longevity, slow population growth rates, low mortality rates, and (at least for the largest) few or no natural predators capable of killing adults. These characteristics, although not exclusive to such megafauna, make them vulnerable to human overexploitation, in part because of their slow population recovery rates.
Evolution of large body size
One observation that has been made about the evolution of larger body size is that rapid rates of increase that are often seen over relatively short time intervals are not sustainable over much longer time periods. In an examination of mammal body mass changes over time, the maximum increase possible in a given time interval was found to scale with the interval length raised to the 0.25 power. This is thought to reflect the emergence, during a trend of increasing maximum body size, of a series of anatomical, physiological, environmental, genetic and other constraints that must be overcome by evolutionary innovations before further size increases are possible. A strikingly faster rate of change was found for large decreases in body mass, such as may be associated with the phenomenon of insular dwarfism. When normalized to generation length, the maximum rate of body mass decrease was found to be over 30 times greater than the maximum rate of body mass increase for a ten-fold change.
In terrestrial mammals

Subsequent to the Cretaceous–Paleogene extinction event that eliminated the non-avian dinosaurs about 66 Ma (million years) ago, terrestrial mammals underwent a nearly exponential increase in body size as they diversified to occupy the ecological niches left vacant. Starting from just a few kg before the event, maximum size had reached ~50 kilograms (110 lb) a few million years later, and ~750 kilograms (1,650 lb) by the end of the Paleocene. This trend of increasing body mass appears to level off about 40 Ma ago (in the late Eocene), suggesting that physiological or ecological constraints had been reached, after an increase in body mass of over three orders of magnitude. However, when considered from the standpoint of rate of size increase per generation, the exponential increase is found to have continued until the appearance of Indricotherium 30 Ma ago. (Since generation time scales with body mass, increasing generation times with increasing size cause the log mass vs. time plot to curve downward from a linear fit.)
Megaherbivores eventually attained a body mass of over 10,000 kilograms (22,000 lb). The largest of these, indricotheres and proboscids, have been hindgut fermenters, which are believed to have an advantage over foregut fermenters in terms of being able to accelerate gastrointestinal transit in order to accommodate very large food intakes. A similar trend emerges when rates of increase of maximum body mass per generation for different mammalian clades are compared (using rates averaged over macroevolutionary time scales). Among terrestrial mammals, the fastest rates of increase of body mass vs. time (in Ma) occurred in perissodactyls (a slope of 2.1), followed by rodents (1.2) and proboscids (1.1), all of which are hindgut fermenters. The rate of increase for artiodactyls (0.74) was about a third of the perissodactyls. The rate for carnivorans (0.65) was slightly lower yet, while primates, perhaps constrained by their arboreal habits, had the lowest rate (0.39) among the mammalian groups studied.
Terrestrial mammalian carnivores from several eutherian groups (the artiodactyl Andrewsarchus – formerly considered a mesonychid, the oxyaenid Sarkastodon, and the carnivorans Amphicyon and Arctodus) all reached a maximum size of about 1,000 kilograms (2,200 lb) (the carnivoran Arctotherium and the hyaenodontid Simbakubwa may have been somewhat larger). The largest known metatherian carnivore, Proborhyaena gigantea, apparently reached 600 kilograms (1,300 lb), also close to this limit. A similar theoretical maximum size for mammalian carnivores has been predicted based on the metabolic rate of mammals, the energetic cost of obtaining prey, and the maximum estimated rate coefficient of prey intake. It has also been suggested that maximum size for mammalian carnivores is constrained by the stress the humerus can withstand at top running speed.
Analysis of the variation of maximum body size over the last 40 Ma suggests that decreasing temperature and increasing continental land area are associated with increasing maximum body size. The former correlation would be consistent with Bergmann's rule, and might be related to the thermoregulatory advantage of large body mass in cool climates, better ability of larger organisms to cope with seasonality in food supply, or other factors; the latter correlation could be explained in terms of range and resource limitations. However, the two parameters are interrelated (due to sea level drops accompanying increased glaciation), making the driver of the trends in maximum size more difficult to identify.
In marine mammals
Since tetrapods (first reptiles, later mammals) returned to the sea in the Late Permian, they have dominated the top end of the marine body size range, due to the more efficient intake of oxygen possible using lungs. The ancestors of cetaceans are believed to have been the semiaquatic pakicetids, no larger than dogs, of about 53 million years (Ma) ago. By 40 Ma ago, cetaceans had attained a length of 20 m (66 ft) or more in Basilosaurus, an elongated, serpentine whale that differed from modern whales in many respects and was not ancestral to them. Following this, the evolution of large body size in cetaceans appears to have come to a temporary halt and then to have backtracked, although the available fossil records are limited. However, in the period from 31 Ma ago (in the Oligocene) to the present, cetaceans underwent a significantly more rapid sustained increase in body mass (a rate of increase in body mass of a factor of 3.2 per million years) than achieved by any group of terrestrial mammals. This trend led to the largest animal of all time, the modern blue whale. Several reasons for the more rapid evolution of large body size in cetaceans are possible. Fewer biomechanical constraints on increases in body size may be associated with suspension in water as opposed to standing against the force of gravity, and with swimming movements as opposed to terrestrial locomotion. Also, the greater heat capacity and thermal conductivity of water compared to air may increase the thermoregulatory advantage of large body size in marine endotherms, although diminishing returns apply.
Among the toothed whales, maximum body size appears to be limited by food availability. Larger size, as in sperm and beaked whales, facilitates deeper diving to access relatively easily-caught, large cephalopod prey in a less competitive environment. Compared to odontocetes, the efficiency of baleen whales' filter feeding scales more favorably with increasing size when planktonic food is dense, making larger sizes more advantageous. The lunge feeding technique of rorquals appears to be more energy efficient than the ram feeding of balaenid whales; the latter technique is used with less dense and patchy plankton. The cooling trend in Earth's recent history may have generated more localities of high plankton abundance via wind-driven upwellings, facilitating the evolution of gigantic whales.
Cetaceans are not the only marine mammals to reach tremendous sizes. The largest mammal carnivorans of all time are marine pinnipeds, the largest of which is the southern elephant seal, which can reach more than 6 m (20 ft) in length and weigh up to 5,000 kg (11,000 lb). Other large pinnipeds include the northern elephant seal at 4,000 kg (8,800 lb), walrus at 2,000 kg (4,400 lb), and Steller sea lion at 1,135 kg (2,502 lb). The sirenians are another group of marine mammals which adapted to fully aquatic life around the same time as the cetaceans did. Sirenians are closely related to elephants. The largest sirenian was the Steller's sea cow, which reached up to 10 m (33 ft) in length and weighed 8,000 to 10,000 kg (18,000 to 22,000 lb), and was hunted to extinction in the 18th century.
In flightless birds

Because of the small initial size of all mammals following the extinction of the non-avian dinosaurs, nonmammalian vertebrates had a roughly ten-million-year-long window of opportunity (during the Paleocene) for evolution of gigantism without much competition. During this interval, apex predator niches were often occupied by reptiles, such as terrestrial crocodilians (e.g. Pristichampsus), large snakes (e.g. Titanoboa) or varanid lizards, or by flightless birds (e.g. Paleopsilopterus in South America). This is also the period when megafaunal flightless herbivorous gastornithid birds evolved in the Northern Hemisphere, while flightless paleognaths evolved to large size on Gondwanan land masses and Europe. Gastornithids and at least one lineage of flightless paleognath birds originated in Europe, both lineages dominating niches for large herbivores while mammals remained below 45 kilograms (99 lb) (in contrast with other landmasses like North America and Asia, which saw the earlier evolution of larger mammals) and were the largest European tetrapods in the Paleocene.
Flightless paleognaths, termed ratites, have traditionally been viewed as representing a lineage separate from that of their small flighted relatives, the Neotropic tinamous. However, recent genetic studies have found that tinamous nest well within the ratite tree, and are the sister group of the extinct moa of New Zealand. Similarly, the small kiwi of New Zealand have been found to be the sister group of the extinct elephant birds of Madagascar. These findings indicate that flightlessness and gigantism arose independently multiple times among ratites via parallel evolution.
Predatory megafaunal flightless birds were often able to compete with mammals in the early Cenozoic. Later in the Cenozoic, however, they were displaced by advanced carnivorans and died out. In North America, the bathornithids Paracrax and Bathornis were apex predators but became extinct by the Early Miocene. In South America, the related phorusrhacids shared the dominant predatory niches with metatherian sparassodonts during most of the Cenozoic but declined and ultimately went extinct after eutherian predators arrived from North America (as part of the Great American Interchange) during the Pliocene. In contrast, large herbivorous flightless ratites have survived to the present.
However, none of the flightless birds of the Cenozoic, including the predatory Brontornis, possibly omnivorous Dromornis stirtoni or herbivorous Aepyornis, ever grew to masses much above 500 kilograms (1,100 lb), and thus never attained the size of the largest mammalian carnivores, let alone that of the largest mammalian herbivores. It has been suggested that the increasing thickness of avian eggshells in proportion to egg mass with increasing egg size places an upper limit on the size of birds. The largest species of Dromornis, D. stirtoni, may have gone extinct after it attained the maximum avian body mass and was then outcompeted by marsupial diprotodonts that evolved to sizes several times larger.
In giant turtles
Giant tortoises were important components of late Cenozoic megafaunas, being present in every nonpolar continent until the arrival of homininans. The largest known terrestrial tortoise was Megalochelys atlas, an animal that probably weighed about 1,000 kg (2,200 lb).
Some earlier aquatic Testudines, e.g. the marine Archelon of the Cretaceous and freshwater Stupendemys of the Miocene, were considerably larger, weighing more than 2,000 kg (4,400 lb).
Megafaunal mass extinctions
Timing and possible causes
Main article: Late Pleistocene extinctions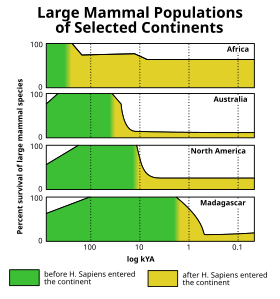
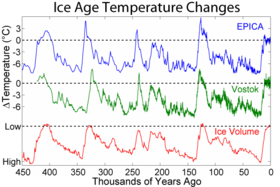
Numerous extinctions occurred during the latter half of the Last Glacial Period when most large mammals went extinct in the Americas, Australia-New Guinea, and Eurasia, including over 80% of all terrestrial animals with a body mass greater than 1,000 kilograms (2,200 lb). Small animals and other organisms like plants were generally unaffected by the extinctions, which is unprecented in previous extinctions during the last 30 million years.
Various theories have attributed the wave of extinctions to human hunting, climate change, disease, extraterrestrial impact, competition from other animals or other causes. However, this extinction near the end of the Pleistocene was just one of a series of megafaunal extinction pulses that have occurred during the last 50,000 years over much of the Earth's surface, with Africa and Asia (where the local megafauna had a chance to evolve alongside modern humans) being comparatively less affected. The latter areas did suffer gradual attrition of megafauna, particularly of the slower-moving species (a class of vulnerable megafauna epitomized by giant tortoises), over the last several million years.
Outside the mainland of Afro-Eurasia, these megafaunal extinctions followed a highly distinctive landmass-by-landmass pattern that closely parallels the spread of humans into previously uninhabited regions of the world, and which shows no overall correlation with climatic history (which can be visualized with plots over recent geological time periods of climate markers such as marine oxygen isotopes or atmospheric carbon dioxide levels). Australia and nearby islands (e.g., Flores) were struck first around 46,000 years ago, followed by Tasmania about 41,000 years ago (after formation of a land bridge to Australia about 43,000 years ago). The role of humans in the extinction of Australia and New Guinea's megafauna has been disputed, with multiple studies showing a decline in the number of species prior to the arrival of humans on the continent and the absence of any evidence of human predation; the impact of climate change has instead been cited for their decline. Similarly, Japan lost most of its megafauna apparently about 30,000 years ago, North America 13,000 years ago and South America about 500 years later, Cyprus 10,000 years ago, the Antilles 6,000 years ago, New Caledonia and nearby islands 3,000 years ago, Madagascar 2,000 years ago, New Zealand 700 years ago, the Mascarenes 400 years ago, and the Commander Islands 250 years ago. Nearly all of the world's isolated islands could furnish similar examples of extinctions occurring shortly after the arrival of humans, though most of these islands, such as the Hawaiian Islands, never had terrestrial megafauna, so their extinct fauna were smaller, but still displayed island gigantism.
An analysis of the timing of Holarctic megafaunal extinctions and extirpations over the last 56,000 years has revealed a tendency for such events to cluster within interstadials, periods of abrupt warming, but only when humans were also present. Humans may have impeded processes of migration and recolonization that would otherwise have allowed the megafaunal species to adapt to the climate shift. In at least some areas, interstadials were periods of expanding human populations.
An analysis of Sporormiella fungal spores (which derive mainly from the dung of megaherbivores) in swamp sediment cores spanning the last 130,000 years from Lynch's Crater in Queensland, Australia, showed that the megafauna of that region virtually disappeared about 41,000 years ago, at a time when climate changes were minimal; the change was accompanied by an increase in charcoal, and was followed by a transition from rainforest to fire-tolerant sclerophyll vegetation. The high-resolution chronology of the changes supports the hypothesis that human hunting alone eliminated the megafauna, and that the subsequent change in flora was most likely a consequence of the elimination of browsers and an increase in fire. The increase in fire lagged the disappearance of megafauna by about a century, and most likely resulted from accumulation of fuel once browsing stopped. Over the next several centuries grass increased; sclerophyll vegetation increased with a lag of another century, and a sclerophyll forest developed after about another thousand years. During two periods of climate change about 120,000 and 75,000 years ago, sclerophyll vegetation had also increased at the site in response to a shift to cooler, drier conditions; neither of these episodes had a significant impact on megafaunal abundance. Similar conclusions regarding the culpability of human hunters in the disappearance of Pleistocene megafauna were derived from high-resolution chronologies obtained via an analysis of a large collection of eggshell fragments of the flightless Australian bird Genyornis newtoni, from analysis of Sporormiella fungal spores from a lake in eastern North America and from study of deposits of Shasta ground sloth dung left in over half a dozen caves in the American Southwest.
Continuing human hunting and environmental disturbance has led to additional megafaunal extinctions in the recent past, and has created a serious danger of further extinctions in the near future (see examples below). Direct killing by humans, primarily for meat or other body parts, is the most significant factor in contemporary megafaunal decline.
A number of other mass extinctions occurred earlier in Earth's geologic history, in which some or all of the megafauna of the time also died out. Famously, in the Cretaceous–Paleogene extinction event, the non-avian dinosaurs and most other giant reptiles were eliminated. However, the earlier mass extinctions were more global and not so selective for megafauna; i.e., many species of other types, including plants, marine invertebrates and plankton, went extinct as well. Thus, the earlier events must have been caused by more generalized types of disturbances to the biosphere.
Consequences of depletion of megafauna
Depletion of herbivorous megafauna results in increased growth of woody vegetation, and a consequent increase in wildfire frequency. Megafauna may help to suppress the growth of invasive plants. Large herbivores and carnivores can suppress the abundance of smaller animals, resulting in their population increase when megafauna are removed.
Effect on nutrient transport
Megafauna play a significant role in the lateral transport of mineral nutrients in an ecosystem, tending to translocate them from areas of high to those of lower abundance. They do so by their movement between the time they consume the nutrient and the time they release it through elimination (or, to a much lesser extent, through decomposition after death). In South America's Amazon Basin, it is estimated that such lateral diffusion was reduced over 98% following the megafaunal extinctions that occurred roughly 12,500 years ago. Given that phosphorus availability is thought to limit productivity in much of the region, the decrease in its transport from the western part of the basin and from floodplains (both of which derive their supply from the uplift of the Andes) to other areas is thought to have significantly impacted the region's ecology, and the effects may not yet have reached their limits. In the sea, cetaceans and pinnipeds that feed at depth are thought to translocate nitrogen from deep to shallow water, enhancing ocean productivity, and counteracting the activity of zooplankton, which tend to do the opposite.
Effect on methane emissions
Large populations of megaherbivores have the potential to contribute greatly to the atmospheric concentration of methane, which is an important greenhouse gas. Modern ruminant herbivores produce methane as a byproduct of foregut fermentation in digestion and release it through belching or flatulence. Today, around 20% of annual methane emissions come from livestock methane release. In the Mesozoic, it has been estimated that sauropods could have emitted 520 million tons of methane to the atmosphere annually, contributing to the warmer climate of the time (up to 10 °C (18 °F) warmer than at present). This large emission follows from the enormous estimated biomass of sauropods, and because methane production of individual herbivores is believed to be almost proportional to their mass.
Recent studies have indicated that the extinction of megafaunal herbivores may have caused a reduction in atmospheric methane. This hypothesis is relatively new. One study examined the methane emissions from the bison that occupied the Great Plains of North America before contact with European settlers. The study estimated that the removal of the bison caused a decrease of as much as 2.2 million tons per year. Another study examined the change in the methane concentration in the atmosphere at the end of the Pleistocene epoch after the extinction of megafauna in the Americas. After early humans migrated to the Americas about 13,000 BP, their hunting and other associated ecological impacts led to the extinction of many megafaunal species there. Calculations suggest that this extinction decreased methane production by about 9.6 million tons per year. This suggests that the absence of megafaunal methane emissions may have contributed to the abrupt climatic cooling at the onset of the Younger Dryas. The decrease in atmospheric methane that occurred at that time, as recorded in ice cores, was 2 to 4 times more rapid than any other decrease in the last half million years, suggesting that an unusual mechanism was at work.
Gallery
Pleistocene extinct megafauna
-
Moa (Dinornis pictured)
-
Diprotodon optatum
-
"Megalania" (Varanus priscus),
-
American lions (Panthera atrox)
-
Woolly mammoth
-
The subfossil lemur Archaeoindris
-
Haast's eagle
-
Restoration of Macrauchenia, a camel-sized member of the extinct ungulate order Litopterna
-
Life restoration of the glyptodonts Doedicurus (front) and Glyptodon
Other extinct Cenozoic megafauna
-
Dromornis stirtoni
-
Asian paraceratheriid rhino Paraceratherium was among the largest land mammals,
-
Reconstructed jaws of megalodon (Otodus megalodon)
-
Deinotherium
-
Kelenken guillermoi
-
Gastornis gigantea
Extant
-
The greater rhea
-
The eastern gorilla
-
Bengal tigers
-
Polar bears
-
The black rhinoceros
-
Unlike woolly rhinos and mammoths, muskoxen narrowly survived the Quaternary extinctions.
-
Hippopotamuses
-
The sperm whale
-
The orca
-
The southern cassowary
-
The common ostrich
-
The saltwater crocodile
-
The Komodo dragon
-
The green anaconda
-
The Chinese giant salamander
-
The giant sunfish
-
The Nile perch
-
The great white shark, the largest macropredatory fish and one of the largest carnivorous shark species, is found worldwide.
-
The manta
-
Examination of a 9 m giant squid
See also
- Australian megafauna
- Bergmann's rule
- Charismatic megafauna
- Cope's rule
- Deep-sea gigantism
- Island gigantism
- Largest organisms
- Largest prehistoric animals
- List of heaviest land mammals
- List of largest mammals
- List of megafauna discovered in modern times
- Megafauna (mythology)
- Megafaunal wolf
- Megaflora
- Megaherb
- Quaternary extinction event
Notes
- Nonavian dinosaur size was not similarly constrained because they had a different relationship between body mass and egg size than birds. The 400 kilograms (880 lb) Aepyornis had larger eggs than nearly all dinosaurs.
- Analysis indicates that 35 genera of North American mammals went extinct more or less simultaneously in this event.
References
- ^ Moleón M, Sánchez-Zapata JA, Donázar JA, Revilla E, Martín-López B, Gutiérrez-Cánovas C, Getz WM, Morales-Reyes Z, Campos-Arceiz A, Crowder LB, Galetti M, González-Suárez M, He F, Jordano P, Lewison R (2020-03-11). "Rethinking megafauna". Proceedings of the Royal Society B: Biological Sciences. 287 (1922): 20192643. doi:10.1098/rspb.2019.2643. hdl:2263/79439. ISSN 0962-8452.
- Malhi Y, Doughty CE, Galetti M, Smith FA, Svenning JC, Terborgh JW (2016-01-26). "Megafauna and ecosystem function from the Pleistocene to the Anthropocene". Proceedings of the National Academy of Sciences. 113 (4): 838–846. Bibcode:2016PNAS..113..838M. doi:10.1073/pnas.1502540113. ISSN 0027-8424. PMC 4743772. PMID 26811442.
- McClenachan L, Cooper AB, Dulvy NK (2016-06-20). "Rethinking Trade-Driven Extinction Risk in Marine and Terrestrial Megafauna". Current Biology. 26 (12): 1640–1646. Bibcode:2016CBio...26.1640M. doi:10.1016/j.cub.2016.05.026. ISSN 1879-0445. PMID 27291051.
- Johnson CN (2002-11-07). "Determinants of loss of mammal species during the Late Quaternary 'megafauna' extinctions: life history and ecology, but not body size". Proceedings of the Royal Society of London. Series B: Biological Sciences. 269 (1506): 2221–2227. doi:10.1098/rspb.2002.2130. ISSN 0962-8452. PMC 1691151. PMID 12427315.
- Barnosky AD (2004-10-01). "Assessing the Causes of Late Pleistocene Extinctions on the Continents". Science. 306 (5693): 70–75. Bibcode:2004Sci...306...70B. CiteSeerX 10.1.1.574.332. doi:10.1126/science.1101476. PMID 15459379. S2CID 36156087.
- Brook BW, Johnson CN (2006). "Selective hunting of juveniles as a cause of the imperceptible overkill of the Australian Pleistocene megafauna". Alcheringa: An Australasian Journal of Palaeontology. 30 (sup1): 39–48. Bibcode:2006Alch...30S..39B. doi:10.1080/03115510609506854. S2CID 84205755.
- ^ Evans AR, Jones D, Boyer AG, Brown JH, Costa DP, Ernest SK, Fitzgerald EM, Fortelius M, Gittleman JL, Hamilton MJ, Harding LE, Lintulaakso K, Lyons SK, Okie JG, Saarinen JJ, Sibly RM, Smith FA, Stephens PR, Theodor JM, Uhen MD (2012-01-30). "The maximum rate of mammal evolution". PNAS. 109 (11): 4187–4190. Bibcode:2012PNAS..109.4187E. doi:10.1073/pnas.1120774109. PMC 3306709. PMID 22308461.
- ^ Smith FA, Boyer AG, Brown JH, Costa DP, Dayan T, Ernest SK, Evans AR, Fortelius M, Gittleman JL, Hamilton MJ, Harding LE, Lintulaakso K, Lyons SK, McCain C, Okie JG, Saarinen JJ, Sibly RM, Stephens PR, Theodor J, Uhen MD (2010-11-26). "The Evolution of Maximum Body Size of Terrestrial Mammals". Science. 330 (6008): 1216–1219. Bibcode:2010Sci...330.1216S. CiteSeerX 10.1.1.383.8581. doi:10.1126/science.1194830. PMID 21109666. S2CID 17272200.
- Clauss M, Frey, R., Kiefer, B., Lechner-Doll, M., Loehlein, W., Polster, C., Roessner, G. E., Streich, W. J. (2003-04-24). "The maximum attainable body size of herbivorous mammals: morphophysiological constraints on foregut, and adaptations of hindgut fermenters" (PDF). Oecologia. 136 (1): 14–27. Bibcode:2003Oecol.136...14C. doi:10.1007/s00442-003-1254-z. PMID 12712314. S2CID 206989975. Archived from the original (PDF) on 2019-06-08. Retrieved 2019-07-13.
- ^ Sorkin B (2008-04-10). "A biomechanical constraint on body mass in terrestrial mammalian predators". Lethaia. 41 (4): 333–347. Bibcode:2008Letha..41..333S. doi:10.1111/j.1502-3931.2007.00091.x.
- Carbone C, Teacher, A, Rowcliffe, J. M. (2007-01-16). "The Costs of Carnivory". PLOS Biology. 5 (2, e22): 363–368. doi:10.1371/journal.pbio.0050022. PMC 1769424. PMID 17227145.
- ^ Ashton KG, Tracy, M. C., de Queiroz, A. (October 2000). "Is Bergmann's Rule Valid for Mammals?". The American Naturalist. 156 (4): 390–415. doi:10.1086/303400. JSTOR 10.1086/303400. PMID 29592141. S2CID 205983729.
- Webb J (2015-02-19). "Evolution 'favours bigger sea creatures'". BBC News. BBC. Archived from the original on 2015-02-22. Retrieved 2015-02-22.
- Heim NA, Knope ML, Schaal EK, Wang SC, Payne JL (2015-02-20). "Cope's rule in the evolution of marine animals". Science. 347 (6224): 867–870. Bibcode:2015Sci...347..867H. doi:10.1126/science.1260065. PMID 25700517. Archived from the original on 2019-07-05. Retrieved 2019-07-13.
- Thewissen JG, Bajpai, S. (1 January 2001). "Whale Origins as a Poster Child for Macroevolution". BioScience. 51 (12): 1037–1049. doi:10.1641/0006-3568(2001)051[1037:WOAAPC]2.0.CO;2. ISSN 0006-3568.
- ^ Goldbogen JA, Cade DE, Wisniewska DM, Potvin J, Segre PS, Savoca MS, Hazen EL, Czapanskiy MF, Kahane-Rapport SR, DeRuiter SL, Gero S, Tønnesen P, Gough WT, Hanson MB, Holt MM, Jensen FH, Simon M, Stimpert AK, Arranz P, Johnston DW, Nowacek DP, Parks SE, Visser F, Friedlaender AS, Tyack PL, Madsen PT, Pyenson ND (2019). "Why whales are big but not bigger: Physiological drivers and ecological limits in the age of ocean giants". Science. 366 (6471): 1367–1372. Bibcode:2019Sci...366.1367G. doi:10.1126/science.aax9044. hdl:10023/19285. PMID 31831666. S2CID 209339266.
- Baker J, Meade A, Pagel M, Venditti C (2015-04-21). "Adaptive evolution toward larger size in mammals". Proceedings of the National Academy of Sciences. 112 (16): 5093–5098. Bibcode:2015PNAS..112.5093B. doi:10.1073/pnas.1419823112. ISSN 0027-8424. PMC 4413265. PMID 25848031.
- Churchill M, Clementz MT, Kohno N (2014-12-19). "Cope's rule and the evolution of body size in Pinnipedimorpha (Mammalia: Carnivora)". Evolution. 69 (1): 201–215. doi:10.1111/evo.12560. ISSN 0014-3820. PMID 25355195.
- Haley MP, Deutsch CJ, Boeuf BJ (April 1991). "A method for estimating mass of large pinnipeds". Marine Mammal Science. 7 (2): 157–164. Bibcode:1991MMamS...7..157H. doi:10.1111/j.1748-7692.1991.tb00562.x. ISSN 0824-0469.
- Goldbogen JA (2018-04-17). "Physiological constraints on marine mammal body size". Proceedings of the National Academy of Sciences. 115 (16): 3995–3997. Bibcode:2018PNAS..115.3995G. doi:10.1073/pnas.1804077115. ISSN 0027-8424. PMC 5910879. PMID 29618615.
- ^ Mitchell KJ, Llamas B, Soubrier J, Rawlence NJ, Worthy TH, Wood J, Lee MS, Cooper A (2014-05-23). "Ancient DNA reveals elephant birds and kiwi are sister taxa and clarifies ratite bird evolution" (PDF). Science. 344 (6186): 898–900. Bibcode:2014Sci...344..898M. doi:10.1126/science.1251981. hdl:2328/35953. PMID 24855267. S2CID 206555952. Archived (PDF) from the original on 2023-03-15. Retrieved 2019-09-24.
- Buffetaut E, Angst D (November 2014). "Stratigraphic distribution of large flightless birds in the Palaeogene of Europe and its palaeobiological and palaeogeographical implications". Earth-Science Reviews. 138: 394–408. Bibcode:2014ESRv..138..394B. doi:10.1016/j.earscirev.2014.07.001.
- Phillips MJ, Gibb GC, Crimp EA, Penny D (January 2010). "Tinamous and moa flock together: mitochondrial genome sequence analysis reveals independent losses of flight among ratites". Systematic Biology. 59 (1): 90–107. doi:10.1093/sysbio/syp079. PMID 20525622.
- Baker AJ, Haddrath O, McPherson JD, Cloutier A (2014). "Genomic Support for a Moa-Tinamou Clade and Adaptive Morphological Convergence in Flightless Ratites". Molecular Biology and Evolution. 31 (7): 1686–1696. doi:10.1093/molbev/msu153. PMID 24825849.
- ^ Murray PF, Vickers-Rich P (2004). Magnificent Mihirungs: The Colossal Flightless Birds of the Australian Dreamtime. Indiana University Press. pp. 51, 314. ISBN 978-0-253-34282-9. Retrieved 7 January 2012.
- Ibid (2004). p. 212. Indiana University Press. ISBN 978-0-253-34282-9.
- Kenneth Carpenter (1999). Eggs, Nests, and Baby Dinosaurs: A Look at Dinosaur Reproduction. Indiana University Press. p. 100. ISBN 978-0-253-33497-8. OCLC 42009424. Retrieved 6 May 2013.
- Jackson FD, Varricchio DJ, Jackson RA, Vila B, Chiappe LM (2008). "Comparison of water vapor conductance in a titanosaur egg from the Upper Cretaceous of Argentina and a Megaloolithus siruguei egg from Spain". Paleobiology. 34 (2): 229–246. doi:10.1666/0094-8373(2008)034[0229:COWVCI]2.0.CO;2. ISSN 0094-8373. S2CID 85880201.
- Ibid (2004). p. 277. Indiana University Press. ISBN 978-0-253-34282-9.
- Hansen DM, Donlan, C. J., Griffiths, C. J., Campbell, K. J. (April 2010). "Ecological history and latent conservation potential: large and giant tortoises as a model for taxon substitutions" (PDF). Ecography. 33 (2): 272–284. Bibcode:2010Ecogr..33..272H. doi:10.1111/j.1600-0587.2010.06305.x. Archived from the original (PDF) on July 24, 2011. Retrieved 2011-02-26.
- Cione AL, Tonni, E. P., Soibelzon, L. (2003). "The Broken Zig-Zag: Late Cenozoic large mammal and tortoise extinction in South America". Rev. Mus. Argentino Cienc. Nat. Nueva Serie. 5 (1): 1–19. doi:10.22179/REVMACN.5.26. ISSN 1514-5158.
- Gordon IJ, Prins HH, Mallon J, Puk LD, Miranda EB, Starling-Manne C, van der Wal R, Moore B, Foley W (2019), Gordon IJ, Prins HH (eds.), "The Ecology of Browsing and Grazing in Other Vertebrate Taxa", The Ecology of Browsing and Grazing II, Cham: Springer International Publishing, pp. 339–404, doi:10.1007/978-3-030-25865-8_15, ISBN 978-3-030-25865-8
- Jaffe AL, Slater GJ, Alfaro ME (2011-08-23). "The evolution of island gigantism and body size variation in tortoises and turtles". Biology Letters. 7 (4): 558–561. doi:10.1098/rsbl.2010.1084. ISSN 1744-9561. PMC 3130210. PMID 21270022.
- Cadena EA, Link A, Cooke SB, Stroik LK, Vanegas AF, Tallman M (December 2021). "New insights on the anatomy and ontogeny of the largest extinct freshwater turtles". Heliyon. 7 (12): e08591. Bibcode:2021Heliy...708591C. doi:10.1016/j.heliyon.2021.e08591. ISSN 2405-8440. PMC 8717240. PMID 35005268.
- Svenning JC, Lemoine RT, Bergman J, Buitenwerf R, Le Roux E, Lundgren E, Mungi N, Pedersen RØ (2024). "The late-Quaternary megafauna extinctions: Patterns, causes, ecological consequences and implications for ecosystem management in the Anthropocene". Cambridge Prisms: Extinction. 2. doi:10.1017/ext.2024.4. ISSN 2755-0958.
- Corlett RT (2006). "Megafaunal extinctions in tropical Asia" (PDF). Tropinet. 17 (3): 1–3. Archived (PDF) from the original on 2016-03-04. Retrieved 2010-10-04.
- Edmeades B. "Megafauna — First Victims of the Human-Caused Extinction". megafauna.com. (internet-published book with Foreword by Paul S. Martin). Archived from the original on 2014-12-25. Retrieved 2020-02-13.
- ^ Martin PS (2005). "Chapter 6. Deadly Syncopation". Twilight of the Mammoths: Ice Age Extinctions and the Rewilding of America. University of California Press. pp. 118–128. ISBN 978-0-520-23141-2. OCLC 58055404. Archived from the original on 2024-03-27. Retrieved 2014-11-11.
- ^ Burney DA, Flannery TF (July 2005). "Fifty millennia of catastrophic extinctions after human contact" (PDF). Trends in Ecology & Evolution. 20 (7): 395–401. doi:10.1016/j.tree.2005.04.022. PMID 16701402. Archived from the original (PDF) on 2010-06-10. Retrieved 2014-11-11.
- Roberts RG, Flannery TF, Ayliffe LK, Yoshida H, Olley JM, Prideaux GJ, Laslett GM, Baynes A, Smith MA, Jones R, Smith BL (2001-06-08). "New Ages for the Last Australian Megafauna: Continent-Wide Extinction About 46,000 Years Ago" (PDF). Science. 292 (5523): 1888–1892. Bibcode:2001Sci...292.1888R. doi:10.1126/science.1060264. PMID 11397939. S2CID 45643228. Archived (PDF) from the original on 2019-02-10. Retrieved 2011-08-26.
- Callaway E (2016-09-21). "Human remains found in hobbit cave". Nature. doi:10.1038/nature.2016.20656. S2CID 89272546.
- Diamond J (2008-08-13). "Palaeontology: The last giant kangaroo". Nature. 454 (7206): 835–836. Bibcode:2008Natur.454..835D. doi:10.1038/454835a. PMID 18704074. S2CID 36583693.
- Turney CS, Flannery TF, Roberts RG, Reid C, Fifield LK, Higham TF, Jacobs Z, Kemp N, Colhoun EA, Kalin RM, Ogle N (2008-08-21). "Late-surviving megafauna in Tasmania, Australia, implicate human involvement in their extinction". PNAS. 105 (34): 12150–12153. Bibcode:2008PNAS..10512150T. doi:10.1073/pnas.0801360105. PMC 2527880. PMID 18719103.
- Roberts R, Jacobs, Z. (October 2008). "The Lost Giants of Tasmania" (PDF). Australasian Science. 29 (9): 14–17. Archived from the original (PDF) on 2011-09-27. Retrieved 2011-08-26.
- ^ Field J, Wroe S, Trueman CN, Garvey J, Wyatt-Spratt S (2013-02-08). "Looking for the archaeological signature in Australian Megafaunal extinctions". Quaternary International. Peopling the last new worlds: the first colonisation of Sahul and the Americas. 285: 76–88. Bibcode:2013QuInt.285...76F. doi:10.1016/j.quaint.2011.04.013. ISSN 1040-6182. Archived from the original on 2012-12-18.
- Dodson J, Field JH (2018). "What does the occurrence of Sporormiella (Preussia) spores mean in Australian fossil sequences?". Journal of Quaternary Science. 33 (4): 380–392. Bibcode:2018JQS....33..380D. doi:10.1002/jqs.3020. ISSN 1099-1417. S2CID 133737405. Archived from the original on 2022-02-14.
- Wroe S, Field JH, Archer M, Grayson DK, Price GJ, Louys J, Faith JT, Webb GE, Davidson I, Mooney SD (2013-09-03). "Reply to Brook et al: No empirical evidence for human overkill of megafauna in Sahul". Proceedings of the National Academy of Sciences. 110 (36): E3369. Bibcode:2013PNAS..110E3369W. doi:10.1073/pnas.1310440110. ISSN 0027-8424. PMC 3767508. PMID 24137797.
- Dortch J, Cupper M, Grün R, Harpley B, Lee K, Field J (2016-08-01). "The timing and cause of megafauna mass deaths at Lancefield Swamp, south-eastern Australia". Quaternary Science Reviews. 145: 161–182. Bibcode:2016QSRv..145..161D. doi:10.1016/j.quascirev.2016.05.042. ISSN 0277-3791. Archived from the original on 2024-03-27.
- Wroe S, Field JH, Archer M, Grayson DK, Price GJ, Louys J, Faith JT, Webb GE, Davidson I, Mooney SD (2013-05-28). "Climate change frames debate over the extinction of megafauna in Sahul (Pleistocene Australia-New Guinea)". Proceedings of the National Academy of Sciences. 110 (22): 8777–8781. Bibcode:2013PNAS..110.8777W. doi:10.1073/pnas.1302698110. ISSN 0027-8424. PMC 3670326. PMID 23650401.
- Norton CJ, Kondo, Y., Ono, A., Zhang, Y., Diab, M. C. (2009-05-23). "The nature of megafaunal extinctions during the MIS 3–2 transition in Japan". Quaternary International. 211 (1–2): 113–122. Bibcode:2010QuInt.211..113N. doi:10.1016/j.quaint.2009.05.002.
- Faith JT, Surovell TA (2009-12-08). "Synchronous extinction of North America's Pleistocene mammals". Proceedings of the National Academy of Sciences. 106 (49): 20641–20645. Bibcode:2009PNAS..10620641F. doi:10.1073/pnas.0908153106. PMC 2791611. PMID 19934040.
- Haynes G (2009). "Introduction to the Volume". In Haynes G (ed.). American Megafaunal Extinctions at the End of the Pleistocene. Vertebrate Paleobiology and Paleoanthropology. Springer. pp. 1–20. doi:10.1007/978-1-4020-8793-6_1. ISBN 978-1-4020-8792-9.
- Fiedel S (2009). "Sudden Deaths: The Chronology of Terminal Pleistocene Megafaunal Extinction". In Haynes G (ed.). American Megafaunal Extinctions at the End of the Pleistocene. Vertebrate Paleobiology and Paleoanthropology. Springer. pp. 21–37. doi:10.1007/978-1-4020-8793-6_2. ISBN 978-1-4020-8792-9.
- Simmons AH (1999). Faunal extinction in an island society: pygmy hippopotamus hunters of Cyprus. Interdisciplinary Contributions to Archaeology. Kluwer Academic/Plenum Publishers. p. 382. doi:10.1007/b109876. ISBN 978-0-306-46088-3. OCLC 41712246. Archived from the original on 2024-03-27. Retrieved 2016-05-07.
- Simmons AH, Mandel, R. D. (December 2007). "Not Such a New Light: A Response to Ammerman and Noller". World Archaeology. 39 (4): 475–482. doi:10.1080/00438240701676169. JSTOR 40026143. S2CID 161791746.
- Steadman DW, Martin PS, MacPhee RD, Jull AJ, McDonald HG, Woods CA, Iturralde-Vinent M, Hodgins GW (2005-08-16). "Asynchronous extinction of late Quaternary sloths on continents and islands". Proc. Natl. Acad. Sci. USA. 102 (33): 11763–11768. Bibcode:2005PNAS..10211763S. doi:10.1073/pnas.0502777102. PMC 1187974. PMID 16085711.
- Cooke SB, Dávalos LM, Mychajliw AM, Turvey ST, Upham NS (2017). "Anthropogenic Extinction Dominates Holocene Declines of West Indian Mammals". Annual Review of Ecology, Evolution, and Systematics. 48 (1): 301–327. doi:10.1146/annurev-ecolsys-110316-022754. S2CID 90558542.
- Anderson A, Sand, C., Petchey, F., Worthy, T. H. (2010). "Faunal extinction and human habitation in New Caledonia: Initial results and implications of new research at the Pindai Caves". Journal of Pacific Archaeology. 1 (1): 89–109. hdl:10289/5404.
- White AW, Worthy, T. H., Hawkins, S., Bedford, S., Spriggs, M. (2010-08-16). "Megafaunal meiolaniid horned turtles survived until early human settlement in Vanuatu, Southwest Pacific". Proc. Natl. Acad. Sci. USA. 107 (35): 15512–15516. Bibcode:2010PNAS..10715512W. doi:10.1073/pnas.1005780107. PMC 2932593. PMID 20713711.
- Burney DA, Burney, L. P., Godfrey, L. R., Jungers, W. L., Goodman, S. M., Wright, H. T., Jull. A. J. T. (July 2004). "A chronology for late prehistoric Madagascar". Journal of Human Evolution. 47 (1–2): 25–63. doi:10.1016/j.jhevol.2004.05.005. PMID 15288523.
- Holdaway RN, Jacomb, C. (2000-03-24). "Rapid Extinction of the Moas (Aves: Dinornithiformes): Model, Test, and Implications". Science. 287 (5461): 2250–2254. Bibcode:2000Sci...287.2250H. doi:10.1126/science.287.5461.2250. PMID 10731144.
- Janoo A (April 2005). "Discovery of isolated dodo bones (Raphus cucullatus (L.), Aves, Columbiformes) from Mauritius cave shelters highlights human predation, with a comment on the status of the family Raphidae Wetmore, 1930". Annales de Paléontologie. 91 (2): 167–180. Bibcode:2005AnPal..91..167J. doi:10.1016/j.annpal.2004.12.002.
- Anderson PK (July 1995). "Competition, Predation, and the Evolution and Extinction of Steller's Sea Cow, Hydrodamalis gigas". Marine Mammal Science. 11 (3): 391–394. Bibcode:1995MMamS..11..391A. doi:10.1111/j.1748-7692.1995.tb00294.x. Archived from the original on 2011-05-11. Retrieved 2011-08-30.
- Cooper A, Turney C, Hughen KA, Brook BW, McDonald HG, Bradshaw CJ (2015-07-23). "Abrupt warming events drove Late Pleistocene Holarctic megafaunal turnover". Science. 349 (6248): 602–6. Bibcode:2015Sci...349..602C. doi:10.1126/science.aac4315. PMID 26250679. S2CID 31686497.
- Müller UC, Pross J, Tzedakis PC, Gamble C, Kotthoff U, Schmiedl G, Wulf S, Christanis K (February 2011). "The role of climate in the spread of modern humans into Europe". Quaternary Science Reviews. 30 (3–4): 273–279. Bibcode:2011QSRv...30..273M. doi:10.1016/j.quascirev.2010.11.016.
- Biello D (2012-03-22). "Big Kill, Not Big Chill, Finished Off Giant Kangaroos". Scientific American news. Archived from the original on 2012-03-23. Retrieved 2012-03-25.
- McGlone M (2012-03-23). "The Hunters Did It". Science. 335 (6075): 1452–1453. Bibcode:2012Sci...335.1452M. doi:10.1126/science.1220176. PMID 22442471. S2CID 36914192.
- ^ Rule S, Brook, B. W., Haberle, S. G., Turney, C. S. M., Kershaw, A. P. (2012-03-23). "The Aftermath of Megafaunal Extinction: Ecosystem Transformation in Pleistocene Australia". Science. 335 (6075): 1483–1486. Bibcode:2012Sci...335.1483R. doi:10.1126/science.1214261. PMID 22442481. S2CID 26675232.
- ^ Johnson CN, Alroy J, Beeton NJ, Bird MI, Brook BW, Cooper A, Gillespie R, Herrando-Pérez S, Jacobs Z, Miller GH, Prideaux GJ, Roberts RG, Rodríguez-Rey M, Saltré F, Turney CS, Bradshaw CJ (10 February 2016). "What caused extinction of the Pleistocene megafauna of Sahul?". Proceedings of the Royal Society B: Biological Sciences. 283 (1824): 20152399. doi:10.1098/rspb.2015.2399. PMC 4760161. PMID 26865301.
- Miller GH, Magee JW, Johnson BJ, Fogel ML, Spooner NA, McCulloch MT, Ayliffe LK (1999-01-08). "Pleistocene Extinction of Genyornis newtoni: Human Impact on Australian Megafauna". Science. 283 (5399): 205–208. doi:10.1126/science.283.5399.205. PMID 9880249.
- Miller G, Magee J, Smith M, Spooner N, Baynes A, Lehman S, Fogel M, Johnston H, Williams D, Clark P, Florian C, Holst R, DeVogel S (2016-01-29). "Human predation contributed to the extinction of the Australian megafaunal bird Genyornis newtoni ~47 ka". Nature Communications. 7: 10496. Bibcode:2016NatCo...710496M. doi:10.1038/ncomms10496. PMC 4740177. PMID 26823193.
- Johnson C (2009-11-20). "Megafaunal Decline and Fall". Science. 326 (5956): 1072–1073. Bibcode:2009Sci...326.1072J. doi:10.1126/science.1182770. PMID 19965418. S2CID 206523763.
- Gill JL, Williams JW, Jackson ST, Lininger KB, Robinson GS (2009-11-20). "Pleistocene Megafaunal Collapse, Novel Plant Communities, and Enhanced Fire Regimes in North America" (PDF). Science. 326 (5956): 1100–1103. Bibcode:2009Sci...326.1100G. doi:10.1126/science.1179504. PMID 19965426. S2CID 206522597. Archived (PDF) from the original on 2017-09-22. Retrieved 2018-11-09.
- Fiedal S (2009). "Sudden Deaths: The Chronology of Terminal Pleistocene Megafaunal Extinction". In Haynes G (ed.). American Megafaunal Extinctions at the End of the Pleistocene. Vertebrate Paleobiology and Paleoanthropology. Springer. pp. 21–37. doi:10.1007/978-1-4020-8793-6_2. ISBN 978-1-4020-8792-9.
- Martin PS (2005). "Chapter 4. Ground Sloths at Home". Twilight of the Mammoths: Ice Age Extinctions and the Rewilding of America. University of California Press. pp. 78–99. ISBN 978-0-520-23141-2. OCLC 58055404. Archived from the original on 2024-03-27. Retrieved 2014-11-11.
- Milman O (February 6, 2019). "The killing of large species is pushing them towards extinction, study finds". The Guardian. Archived from the original on February 7, 2019. Retrieved February 13, 2019.
- Ripple WJ, et al. (2019). "Are we eating the world's megafauna to extinction?". Conservation Letters. 12 (3): e12627. Bibcode:2019ConL...12E2627R. doi:10.1111/conl.12627.
- Alroy J (2008-08-12). "Dynamics of origination and extinction in the marine fossil record". PNAS. 105 Suppl 1 (Supplement_1): 11536–11542. Bibcode:2008PNAS..10511536A. doi:10.1073/pnas.0802597105. PMC 2556405. PMID 18695240.
- D'Hondt S (2005-12-01). "Consequences of the Cretaceous/Paleogene Mass Extinction for Marine Ecosystems". Annual Review of Ecology, Evolution, and Systematics. 36 (1): 295–317. doi:10.1146/annurev.ecolsys.35.021103.105715. ISSN 1543-592X.
- ^ Malhi Y, Doughty CE, Galetti M, Smith FA, Svenning JC, Terborgh JW (2016-01-26). "Megafauna and ecosystem function from the Pleistocene to the Anthropocene". Proceedings of the National Academy of Sciences. 113 (4): 838–846. doi:10.1073/pnas.1502540113. ISSN 0027-8424. PMC 4743772. PMID 26811442.
- Johnson C (2009-07-22). "Ecological consequences of Late Quaternary extinctions of megafauna". Proceedings of the Royal Society B: Biological Sciences. 276 (1667): 2509–2519. doi:10.1098/rspb.2008.1921. ISSN 0962-8452. PMC 2684593. PMID 19324773.
- Mungi NA, Jhala YV, Qureshi Q, le Roux E, Svenning JC (October 2023). "Megaherbivores provide biotic resistance against alien plant dominance". Nature Ecology & Evolution. 7 (10): 1645–1653. doi:10.1038/s41559-023-02181-y. ISSN 2397-334X.
- Wolf A, Doughty CE, Malhi Y (2013). "Lateral Diffusion of Nutrients by Mammalian Herbivores in Terrestrial Ecosystems". PLoS ONE. 8 (8): e71352. Bibcode:2013PLoSO...871352W. doi:10.1371/journal.pone.0071352. PMC 3739793. PMID 23951141.
- Marshall M (2013-08-11). "Ecosystems still feel the pain of ancient extinctions". New Scientist. Archived from the original on 2015-07-04. Retrieved 2013-08-12.
- ^ Doughty CE, Wolf A, Malhi Y (2013-08-11). "The legacy of the Pleistocene megafauna extinctions on nutrient availability in Amazonia". Nature Geoscience. 6 (9): 761–764. Bibcode:2013NatGe...6..761D. doi:10.1038/ngeo1895.
- Roman J, McCarthy J (2010). "The Whale Pump: Marine Mammals Enhance Primary Productivity in a Coastal Basin". PLOS ONE. 5 (10): e13255. Bibcode:2010PLoSO...513255R. doi:10.1371/journal.pone.0013255. PMC 2952594. PMID 20949007.
- ^ Wilkinson DM, Nisbet, E. G., Ruxton, G. D. (2012-05-08). "Could methane produced by sauropod dinosaurs have helped drive Mesozoic climate warmth?". Current Biology. 22 (9): R292 – R293. Bibcode:2012CBio...22.R292W. doi:10.1016/j.cub.2012.03.042. PMID 22575462.
- "Dinosaur gases 'warmed the Earth'". BBC Nature News. 2012-05-07. Archived from the original on 2015-12-01. Retrieved 2012-05-08.
- ^ Smith FA, Elliot, S. M., Lyons, S. K. (2010-05-23). "Methane emissions from extinct megafauna". Nature Geoscience. 3 (6): 374–375. Bibcode:2010NatGe...3..374S. doi:10.1038/ngeo877.
- Kelliher FM, Clark, H. (2010-03-15). "Methane emissions from bison—An historic herd estimate for the North American Great Plains". Agricultural and Forest Meteorology. 150 (3): 473–577. Bibcode:2010AgFM..150..473K. doi:10.1016/j.agrformet.2009.11.019.
- Tsubamoto T (2012). "Estimating body mass from the astragalus in mammals". Acta Palaeontologica Polonica: 259–265. doi:10.4202/app.2011.0067. S2CID 54686160.
- Stuart AJ (November 1991). "Mammalian extinctions in the Late Pleistocene of northern Eurasia and North America". Biological Reviews. 66 (4): 453–562. doi:10.1111/j.1469-185X.1991.tb01149.x. PMID 1801948. S2CID 41295526.
- Sample, Ian (19 February 2010). "Great white shark is more endangered than tiger, claims scientist". The Guardian. Archived from the original on 9 September 2013. Retrieved 14 August 2013.