History
Photopharmacology is an emerging multidisciplinary field that combines photochemistry and pharmacology. Built upon the ability of light to change the pharmacokinetics and pharmacodynamics of bioactive molecules, it aims at regulating the activity of drugs in vivo by using light. The light-based modulation is achieved by incorporating molecular photoswitches such as azobenzene and diarylethenes or photocages such as o-nitrobenzyl, coumarin, and BODIPY compounds into the pharmacophore. This selective activation of the biomolecules helps prevent or minimize off-target activity and systemic side effects. Moreover, light being the regulatory element offers additional advantages such as the ability to be delivered with high spatiotemporal precision, low to negligible toxicity, and the ability to be controlled both qualitatively and quantitatively by tuning its wavelength and intensity.
Though photopharmacology is a relatively new field, the concept of using light in therapeutic applications came into practice a few decades ago. Photodynamic therapy (PDT) is a well-established clinically practiced protocol in which photosensitizers are used to produce singlet oxygen for destroying diseased or damaged cells or tissues. Optogenetics is another method that relies on light for dynamically controlling biological functions especially brain and neural. Though this approach has proven useful as a research tool, its clinical implementation is limited by the requirement for genetic manipulation. Mainly, these two techniques laid the foundation for photopharmacology. Today, it is a rapidly evolving field with diverse applications in both basic research and clinical medicine which has the potential to overcome some of the challenges limiting the range of applications of the other light-guided therapies.
Figure 1. Schematic representation of the mechanism of (a) photopharmacology (b) photodynamic therapy, and (c) optogenetics.
The discovery of natural photoreceptors such as rhodopsins in the eye inspired the biomedical and pharmacology research community to engineer light-sensitive proteins for therapeutic applications. The development of synthetic photoswitchable molecules is the most significant milestone in the history of light-delivery systems. Scientists are continuing with their efforts to explore new photoswitches and delivery strategies with enhanced performance to target different biological molecules such as ion channels, nucleic acid, and enzyme receptors. Photopharmacology research progressed from in vitro to in vivo studies in a significantly short period of time yielding promising results in both forms. Clinical trials are underway to assess the safety and efficacy of these photopharmacological therapies further and validate their potential as an innovative drug delivery approach.
Mechanism of action
Molecular photoswitches are utilized in the field of photopharmacology, where the energetics of a molecule can be reversibly controlled with light to achieve spatial and temporal resolution of a particular effect. Photoswitches may function by undergoing photoisomerization through which light is used to conformationally adapt a molecule to a biological site, or through an environmental effect where an external factor such as a solvent effect or hydrogen bonding can selectively allow or quench an emissive state within a molecule. To visualize photophysical processes, a useful depiction is the Jablonski diagram. This is a diagram which depicts electronic and vibrational energy levels within a molecule as vertical levels and shows the possible relaxation pathways from excited states. Typically, the ground state is referred to as S0, and is drawn at the bottom of the figure with nearby vibrational excitations just above it. An absorption will promote an electron into the S1 state at any vibrational energy level, or into a higher order excited state if the absorbed energy has enough magnitude. The excited state can then undergo internal conversion which is the electronic relaxation to a lower state with the same vibrational energetics or vibrational relaxation within a state. This may be followed by an intersystem crossing wherein the electron undergoes a spin flip, or a radiative or nonradiative decay back to the ground state.

One example of an organic compound that undergoes photoisomerization is azobenzene. The structure is two phenyl rings joined with a N=N double bond and is the simplest aryl azo compound. Azobenzene and its derivatives have two accessible absorbance bands: the S1 state from a n-π* transition which can be excited into using blue light, and the S2 state from a π-π* transition that can be excited into using ultraviolet light. Azobenzene and its derivatives have two isomers, trans and cis. The trans isomer, having the phenyl rings on opposite sides of the azo double bond, is the thermally preferred isomer as there is less stereoelectronic distortion and more delocalization present. However, excitation of the trans isomer to the S2 state facilitates a shift to the cis isomer. The S1 absorption is associated with a conversion back to the trans isomer. In this way, azobenzene and its derivatives can act as reversible stores of energy by maintaining a strained configuration in the cis isomer. Modifications of the substituents on azobenzene allow the energetics of these absorptions to be tuned, and if they are engineered such that the two absorption bands overlap a single wavelength of light can be used to flip between them. There are a number of similar photoswitches which isomerize between E and Z configurations across an azo group (for instance, azobenzene and azopyrazole) or an ethylene bridge (for instance, stilbene and hemithioindigo).

Alternatively, photoswitches may themselves be emissive and exhibit environmental control over their properties. One such example is a class of ruthenium polypyridyl coordination complexes. Typically they contain two bidentate bipyridine or phenanthroline ligands and an extended phenanthroline-phenazine bidentate ligand such as dipyridophenazine (dppz). These complexes have an accessible metal to ligand charge transfer excited state (MLCT) which undergoes rapid intersystem crossing to a MLCT state due to the strong spin-orbit coupling of the ruthenium center. These excited states are localized on the extended ligand phenazine nitrogens, and emission occurs from the MLCT state. Hydrogen bonding interactions such as the presence of water around these nitrogen atoms stabilizes the MLCT state, quenching the emission process. Thus, by controlling whether an aqueous or otherwise protic polar solvent is present, emissive behaviors can be “turned on/off”, and alternation between “bright states” and “dark states” is facilitated. This light switch behavior makes these and similar complexes of recent interest in photopharmacological applications such as photodynamic therapy.
Molecules
As previously mentioned, photopharmacology relies on the use of molecular photoswitches being incorporated into the structure of biologically active molecules which allows their potency to be controlled optically. They are introduced into the structure of bioactive compounds via insertion, extension, or bioisosteric replacement. These incorporations can be supported by structural considerations of the molecule or SAR (structure-activity relationship) analysis to determine the optimal position. Some examples of photoswitchable molecules commonly used in photopharmacology are azobenzenes, diarylethenes, and photocages.
Azobenzenes
Azobenzenes are a class of photoswitchable molecules and are used in photopharmalogical applications for their reversible photoisomerization, as described in the previous section. An example of a photoswitchable molecule that uses azobenzene is phototrexate. Phototrexate is an inhibitor of human dihydrofolate reductase and is an analogue of methotrexate, a chemotherapy agent. When in its photoactive cis form, phototrexate has been shown to be a potent antifolate and is relatively inactive when in the trans form. The azologization, or incorporation of azobenzene, of methotrexate allows for control of cytotoxic activity and is considered a step forward in developing targeted anticancer drugs with localized efficacy.
Diarylethenes
Diarylethene photoswitches have reversible cyclization and cycloreversion reactions that are photoinduced. They are a class of compounds that have aromatic functional groups bonded to each end of a carbon-carbon double bond. An example of this class of molecule that is used in photopharmacology is stilbene. Under the influence of light, stilbene switches between its two isomers (E and Z).
Figure 4. Figure showing stilbene isomerizations under light from E to Z.
Diarylethenes have been shown to have some advantages over the more researched azobenzenes switches, such as thermal irreversibility, high photoswitching efficiency, favorable cellular stability, and low toxicity. Diarylethenes have been shown to have promise in fields other than photopharmacology as well. These fields include optical data storage, optoelectronic devices, supramolecular self-assembly and anti-counterfeiting.
Photocages
A class of substances known as photocages contain “photosensitive groups, also known as 'photoremovable protecting groups", from which target substances are released upon exposure to specific wavelengths of light”. The photosensitive groups physically and chemically protect the target from being released until the molecule undergoes photoreaction. Due to these interactions with light, they are commonly used molecules in photopharmacology. More recently, they have played an important role in photoactivated chemotherapy (PACT). In PACT, photocages utilize a photoremovable protecting group that protects cytotoxic drugs until the bond is cleaved via light interaction and the cytotoxic drug is released. Some well-known photocages include “o-nitrobenzyl derivatives, coumarin derivatives, BODIPY, xanthene derivatives, quinone and diarylenes derivatives”. However, there are limitations with using photocages in clinical applications as there are not many PPGs that can be used in vivo. This is due to PPG-payload conjugates needing to have acceptable solubility and biological inertness for biocompatibility and the need for efficient uncaging above 600 nm.
Figure 5. Example of a photocage release system activated by NIR.
Application
Photopharmacology, the use of light to control the activity of drugs, has emerged as a promising approach to drug delivery and therapy. By harnessing the power of light, researchers can achieve precise control over drug release and activation, offering new possibilities for targeted and personalized treatments. This subsection explores the application of photopharmacology in drug delivery, focusing on recent advancements and potential clinical applications.
In one study, researchers designed HDAC inhibitors which can be activated or deactivated with light, providing precise therapeutic control. This approach could reduce the side effects of traditional chemotherapy by targeting inhibitors to specific body areas, potentially leading to more effective and personalized cancer treatments.
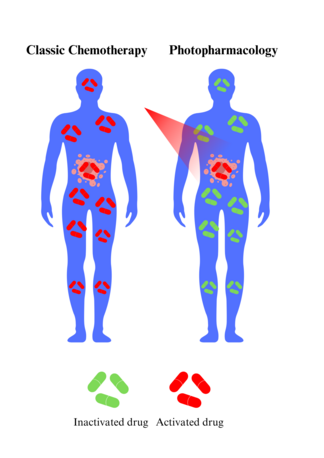
In another study, the researchers developed a strategy to attach a photoswitchable group to a common antibiotic; ciprofloxacin. By attaching the photoswitchable group, researchers can control the activity of ciprofloxacin with light. This approach could potentially lead to new ways of treating bacterial infections, with the ability to switch the antibiotic's activity on and off as needed. In this paper an in vitro protocol to test different light wavelengths on human cancer cell lines is developed, finding that blue light most effectively inhibited cell growth. This suggests that photopharmacology could offer new cancer treatment options by targeting specific light wavelengths to modulate drug activity in tumor cells.
Another application of photopharmacology is developing a luminescent photoCORM grafted on carboxymethyl chitosan, which, when exposed to light, releases carbon monoxide (CO) to induce apoptotic death in colorectal cancer cells, demonstrating precise control over CO release for targeted cancer therapy.
Researchers developed a toolbox of photoswitchable antagonists that can interact with GPCRs, a class of proteins involved in various cellular processes. By using light to switch the activity of these antagonists, researchers can control the interaction between the antagonists and GPCRs in real time. This approach allows for precise modulation of GPCR activity, which could lead to new insights into cellular signaling pathways and potential therapeutic applications.
In another application by using light to control the assembly of nanopores, researchers can potentially regulate the flow of ions or molecules through these nanopores. This approach could have applications in various fields, including sensing, drug delivery, and nanotechnology.
Another paper reports on the use of photopharmacology to control drug activity; multifunctional fibers in the study deliver light and drugs to specific body areas. Implanted fibers activate light-responsive drugs, altering their structure, and offering precise drug delivery for conditions needing exact timing or dosage.
In another study, ligands were designed to switch their binding mode to G-quadruplex DNA upon exposure to visible light. This method could potentially modulate the activity of G-quadruplex DNA, crucial in gene expression and telomere maintenance, offering new therapeutic avenues, particularly in cancer treatment. The study underscores photopharmacology's promise in targeting specific DNA structures, suggesting G-quadruplex DNA as a viable target for future photopharmacological interventions.
Another study developed photoactivatable antibody-photoCORM conjugates targeting human ovarian cancer cells, releasing CO upon light exposure to diminish cell viability. This approach offers precise cancer cell targeting while minimizing harm to healthy tissue, showcasing the potential of photopharmacology in cancer therapy.
In another paper, a photoactivatable compound that binds to and modulates the activity of the CRY1 protein, regulating the mammalian circadian clock, was developed. By using light to control the compound's activity, researchers can potentially treat circadian rhythm disorders and related health conditions by modulating the function of CRY1. Photopharmacology involves using light to control the activity of drugs.
In another application researchers used photopharmacology to control drug release and focus on a drug interacting with tubulin, visualizing its release in real time with time-resolved serial crystallography. This technique offers insights into drug-tubulin interactions and demonstrates the potential for designing drugs with precise actions.
Future directions
The future of photopharmacology holds immense promise. It has the potential to revolutionize conventional drug therapy offering new avenues for precision medicine, treating neurological disorders, and in the field of oncology and ophthalmology. Additionally, it holds promise for the field of regenerative medicine where photoswitches can be used to modulate the activity of signaling pathways for targeted tissue repair and regeneration.
Photopharmacology will continue to grow and expand with the new discoveries and advances happening in other related fields such as synthetic chemistry, biology, nanotechnology, pharmacology, and bioengineering. While the potential of photopharmacology is vast, some challenges must be addressed to make it a clinical reality. One challenge is the development of stable and biocompatible photoswitches that are selective for their target receptors without cross-activity. It is particularly important that these photoswitchables have their absorbance bands fall within the wavelength range of 650 nm to 900 nm. Hence, optimum molecular designing of photoswitches is required to achieve the characteristics mentioned above and desired level of performance. At present, photopharmacology uses a rational drug design approach based on studying the structure-activity relationship, however, a phenotypic screening for photoswitchable drugs could also be beneficial.
In order to achieve good spatial-temporal control over drug activity there should be a significant difference between the activity of isomers. However, understanding the structural changes during the biological effects induced by photoswitching is limited. This scarcity of knowledge is also a challenge for the growth of this field, as it hampers the optimization of the activity and potency of the isomers to obtain the expected outcomes during applications.
The biggest challenge in photopharmacology is finding appropriate and effective ways to deliver light to deep tissues in the body and tissues avoiding issues such as scattering and absorption. Various strategies have been attempted in this regard, one being the development of photoswitchable ligands that respond to deep-tissue penetrating wavelengths like red or infrared light. Moreover, some recent preclinical studies have spurred the development of wireless, compact or injectable, and remotely controllable devices capable of delivering light to neural tissues with minimal damage. There are novel optofluidic systems that can simultaneously regulate both drug delivery and light activity at specific sites. Although external delivery of light is the most preferred method, the use of internal exogenous light sources such as luminescent compounds where light would be delivered directly at the site of action. This could avoid the issues related to light penetration and also enhance the degree of selectivity. In addition, this creates the opportunity to use photopharmacology as a theranostic approach that combines targeted drug delivery and molecular imaging.
References
- ^ Hüll, Katharina; Morstein, Johannes; Trauner, Dirk (2018-11-14). "In Vivo Photopharmacology". Chemical Reviews. 118 (21): 10710–10747. doi:10.1021/acs.chemrev.8b00037. ISSN 0009-2665. PMID 29985590.
- ^ Lerch, Michael M.; Hansen, Mickel J.; van Dam, Gooitzen M.; Szymanski, Wiktor; Feringa, Ben L. (2016-09-05). "Emerging Targets in Photopharmacology". Angewandte Chemie International Edition. 55 (37): 10978–10999. doi:10.1002/anie.201601931. ISSN 1433-7851. PMID 27376241.
- ^ Arkhipova, Valentina; Fu, Haigen; Hoorens, Mark W. H.; Trinco, Gianluca; Lameijer, Lucien N.; Marin, Egor; Feringa, Ben L.; Poelarends, Gerrit J.; Szymanski, Wiktor; Slotboom, Dirk J.; Guskov, Albert (2021-01-27). "Structural Aspects of Photopharmacology: Insight into the Binding of Photoswitchable and Photocaged Inhibitors to the Glutamate Transporter Homologue". Journal of the American Chemical Society. 143 (3): 1513–1520. doi:10.1021/jacs.0c11336. ISSN 0002-7863. PMC 7844824. PMID 33449695.
- ^ Velema, Willem A.; Szymanski, Wiktor; Feringa, Ben L. (2014-02-12). "Photopharmacology: Beyond Proof of Principle". Journal of the American Chemical Society. 136 (6): 2178–2191. doi:10.1021/ja413063e. ISSN 0002-7863. PMID 24456115.
- Lakowicz, Joseph R. Principles of Fluorescence Spectroscopy, 3rd Edition (2006), Page 5, Springer Science+Business Media, LLC. ISBN 978-0 387-31278-1.
- Qiao, Zhi., et. al. Azobenzene-isoxazoline as photopharmacological ligand for optical control of insect GABA receptor and behavior. Pest Manag. Sci. 78, 467–474 (2022).
- ^ Kobauri, Piermichele; Dekker, Frank J.; Szymanski, Wiktor; Feringa, Ben L. (2023). "Rational Design in Photopharmacology with Molecular Photoswitches". Angewandte Chemie International Edition. 62 (30): e202300681. doi:10.1002/anie.202300681. PMID 37026576.
- Andrew W., Lincoln, Per., Tuite, Eimer M. Environmental effects on the photophysics of transition metal complexes with dipyridophenazine (dppz) and related ligands. Coordination Chemistry Reviews 255, 2676–2692 (2011).
- Arkhipova, Valentina; Fu, Haigen; Hoorens, Mark W. H.; Trinco, Gianluca; Lameijer, Lucien N.; Marin, Egor; Feringa, Ben L.; Poelarends, Gerrit J.; Szymanski, Wiktor; Slotboom, Dirk J.; Guskov, Albert (2021). "Structural Aspects of Photopharmacology: Insight into the Binding of Photoswitchable and Photocaged Inhibitors to the Glutamate Transporter Homologue". Journal of the American Chemical Society. 143 (3): 1513–1520. doi:10.1021/jacs.0c11336. PMID 33449695.
- ^ Matera, Carlo; Gomila, Alexandre M. J.; Camarero, Núria; Libergoli, Michela; Soler, Concepció; Gorostiza, Pau (2018). "Photoswitchable Antimetabolite for Targeted Photoactivated Chemotherapy". Journal of the American Chemical Society. 140 (46): 15764–15773. doi:10.1021/jacs.8b08249. hdl:2445/126377. PMID 30346152.
- ^ Li, Ziyong; Zeng, Xiaoyan; Gao, Caimin; Song, Jinzhao; He, Fan; He, Tian; Guo, Hui; Yin, Jun (2023). "Photoswitchable diarylethenes: From molecular structures to biological applications". Coordination Chemistry Reviews. 497. doi:10.1016/j.ccr.2023.215451.
- ^ Li, Yajing; Wang, Maolin; Wang, Fang; Lu, Sheng; Chen, Xiaoqiang (2023). "Recent progress in studies of photocages". Smart Molecules. 1. doi:10.1002/smo.20220003.
- ^ Egyed, Alexandra; Németh, Krisztina; Molnár, Tibor Á.; Kállay, Mihály; Kele, Péter; Bojtár, Márton (2023). "Turning Red without Feeling Embarrassed─Xanthenium-Based Photocages for Red-Light-Activated Phototherapeutics". Journal of the American Chemical Society. 145 (7): 4026–4034. doi:10.1021/jacs.2c11499. PMC 9951246. PMID 36752773.
- Black, Caroline E.; Zhou, Eugene; Deangelo, Caitlin M.; Asante, Isaac; Louie, Stan G.; Petasis, Nicos A.; Humayun, Mark S. (2020). "Cyanine Nanocages Activated by Near-Infrared Light for the Targeted Treatment of Traumatic Brain Injury". Frontiers in Chemistry. 8: 769. Bibcode:2020FrCh....8..769B. doi:10.3389/fchem.2020.00769. PMC 7489144. PMID 33062635.
- Szymanski, Wiktor; Ourailidou, Maria E.; Velema, Willem A.; Dekker, Frank J.; Feringa, Ben L. (2015). "Light-controlled histone deacetylase (HDAC) inhibitors: towards photopharmacological chemotherapy". Chemistry: A European Journal. 21 (46): 16517–16524. doi:10.1002/chem.201502809. PMC 5221732. PMID 26418117.
- Velema, Willem A.; Hansen, Mickel J.; Lerch, Michael M.; Driessen, Arnold J. M.; Szymanski, Wiktor; Feringa, Ben L. (2015). "Ciprofloxacin–photoswitch conjugates: a facile strategy for photopharmacology" (PDF). Bioconjugate Chemistry. 26 (12): 2592–2597. doi:10.1021/acs.bioconjchem.5b00591. PMID 26574623.
- Hopkins, S. L.; Siewert, B.; Askes, S. H. C.; Veldhuizen, P.; Zwier, R.; Heger, Michal; Bonnet, Sylvestre (2016). "An in vitro cell irradiation protocol for testing photopharmaceuticals and the effect of blue, green, and red light on human cancer cell lines". Photochemical & Photobiological Sciences. 15 (5): 644–653. doi:10.1039/c5pp00424a. PMC 5044800. PMID 27098927.
- Chakraborty, Indranil; Jimenez, Jorge; Mascharak, P. K. (2017). "CO-Induced apoptotic death of colorectal cancer cells by a luminescent photoCORM grafted on biocompatible carboxymethyl chitosan". Chemical Communications. 53 (40): 5519–5522. doi:10.1039/c7cc02842c. PMC 5728991. PMID 28466932.
- Hauwert, Niels J.; Mocking, Tamara A. M.; Da Costa Pereira, Daniel; Kooistra, Albert J.; Wijnen, Lisa M.; Vreeker, Gerda C. M.; Verweij, Eléonore W. E.; De Boer, Albertus H.; Smit, Martine J.; De Graaf, Chris; Vischer, Henry F.; De Esch, Iwan J. P.; Wijtmans, Maikel; Leurs, Rob (2018). "Synthesis and characterization of a bidirectional photoswitchable antagonist toolbox for real-time GPCR photopharmacology". Journal of the American Chemical Society. 140 (12): 4232–4243. doi:10.1021/jacs.7b11422. PMC 5879491. PMID 29470065.
- Mutter, Natalie L.; Volarić, Jana; Szymanski, Wiktor; Feringa, Ben L.; Maglia, Giovanni (2019). "Reversible photocontrolled nanopore assembly". Journal of the American Chemical Society. 141 (36): 14356–14363. doi:10.1021/jacs.9b06998. PMC 6743218. PMID 31469268.
- Frank, James A.; Antonini, Marc-Joseph; Chiang, Po-Han; Canales, Andres; Konrad, David B.; Garwood, Indie C.; Rajic, Gabriela; Koehler, Florian; Fink, Yoel; Anikeeva, Polina (2020). "In vivo photopharmacology enabled by multifunctional fibers". ACS Chemical Neuroscience. 11 (22): 3802–3813. doi:10.1021/acschemneuro.0c00577. PMC 10251749. PMID 33108719.
- o'Hagan, Michael P.; Ramos-Soriano, Javier; Haldar, Susanta; Sheikh, Sadiyah; Morales, Juan C.; Mulholland, Adrian J.; Galan, M. Carmen (2020). "Visible-light photoswitching of ligand binding mode suggests G-quadruplex DNA as a target for photopharmacology". Chemical Communications. 56 (38): 5186–5189. doi:10.1039/d0cc01581d. hdl:1983/51860d75-af8d-4d5b-ae49-b7e8a1282d12. PMID 32267261.
- Kawahara, Brian; Gao, Lucy; Cohn, Whitaker; Whitelegge, Julian P.; Sen, Suvajit; Janzen, Carla; Mascharak, Pradip K. (2020). "Diminished viability of human ovarian cancer cells by antigen-specific delivery of carbon monoxide with a family of photoactivatable antibody-photoCORM conjugates". Chemical Science. 11 (2): 467–473. doi:10.1039/c9sc03166a. PMC 7067254. PMID 32190266.
- Kolarski, Dušan; Miller, Simon; Oshima, Tsuyoshi; Nagai, Yoshiko; Aoki, Yugo; Kobauri, Piermichele; Srivastava, Ashutosh; Sugiyama, Akiko; Amaike, Kazuma; Sato, Ayato; Tama, Florence; Szymanski, Wiktor; Feringa, Ben L.; Itami, Kenichiro; Hirota, Tsuyoshi (2021). "Photopharmacological manipulation of mammalian CRY1 for regulation of the circadian clock". Journal of the American Chemical Society. 143 (4): 2078–2087. doi:10.1021/jacs.0c12280. PMC 7863067. PMID 33464888.
- Wranik, Maximilian; et al. (2023). "Watching the release of a photopharmacological drug from tubulin using time-resolved serial crystallography". Nature Communications. 14: 903. Bibcode:2023NatCo..14..903W. doi:10.1038/s41467-023-36481-5. hdl:11585/966526.
- Berizzi, Alice E.; Goudet, Cyril (2020), "Strategies and considerations of G-protein-coupled receptor photopharmacology", From Structure to Clinical Development: Allosteric Modulation of G Protein-Coupled Receptors, Advances in Pharmacology, vol. 88, Elsevier, pp. 143–172, doi:10.1016/bs.apha.2019.12.001, ISBN 978-0-12-820187-9, PMID 32416866, retrieved 2024-04-15