Revision as of 05:27, 22 May 2016 editEdChem (talk | contribs)Autopatrolled, Extended confirmed users, Pending changes reviewers17,226 edits →Ionic systems: fix broken ref← Previous edit | Revision as of 14:48, 22 May 2016 edit undoJonesey95 (talk | contribs)Autopatrolled, Extended confirmed users, Page movers, Mass message senders, Template editors375,969 editsm Fixing citation DOI or LCCN or arXiv parameter error or Cite DOI template using AutoEdNext edit → | ||
Line 26: | Line 26: | ||
:] | :] | ||
In a ], an ] is combined with an ] to generate a ], and is a specific example of a ] to a form a ] five-membered aromatic rings with ] and ].<ref>{{cite journal|title = 1.3-Dipolare Cycloadditionen Rückschau und Ausblick|first = Rolf|last = Huisgen|authorlink = Rolf Huisgen|journal = ]|volume = 75|year = 1963|pages = 604–637|doi = 10.1002/ange. |
In a ], an ] is combined with an ] to generate a ], and is a specific example of a ] to a form a ] five-membered aromatic rings with ] and ].<ref>{{cite journal|title = 1.3-Dipolare Cycloadditionen Rückschau und Ausblick|first = Rolf|last = Huisgen|authorlink = Rolf Huisgen|journal = ]|volume = 75|year = 1963|pages = 604–637|doi = 10.1002/ange.19630751304|language = German}}</ref> American ] ] has described this ] as "the cream of the crop" of ]<ref>{{cite journal|first1 = H. C.|last1 = Kolb|first2 = M. G.|last2 = Finn|first3 = K. B.|last3 = Sharpless|authorlink3 = K. Barry Sharpless|title = Click Chemistry: Diverse Chemical Function from a Few Good Reactions|year = 2001|journal = ]|volume = 40|issue = 11|pages = 2004–2021|doi = 10.1002/1521-3773(20010601)40:11<2004::AID-ANIE2004>3.0.CO;2-5}}</ref> and "the premier example of a click reaction."<ref>{{cite journal|last1 = Kolb|first1 = H. C.|last2 = Sharpless|first2 = B. K.|authorlink2 = K. Barry Sharpless|title = The Growing Impact of Click Chemistry on Drug Discovery|year = 2003|volume = 8|issue = 24|pages = 1128–1137|doi=10.1016/S1359-6446(03)02933-7|journal = ]}}</ref> | ||
:] | :] | ||
Revision as of 14:48, 22 May 2016
Aromatization is a chemical reaction in which an aromatic system is formed. It can also refer to the production of a new aromatic moiety in a molecule which is already aromatic. Theoretically, this can be achieved by dehydrogenation of existing cyclic compounds (such as in converting cyclohexane into benzene) or by formation of new cyclic system (such as in the cyclotrimerization of acetylene to benzene). Practically, other moieties are typically required to carry out such conversion, and other approaches like applying condensation reactions are possible. Aromatization includes the formation of any aromatic system (including heterocyclic systems), and is not restricted to benzene and its derivatives.
Formation of new cyclic systems
Alkyne cyclotrimerizations

Many aromatization processes start with two or three acyclic molecules which are fused into a new aromatic ring. Alkyne trimerization is one simple case whereby the cycloaddition of three alkynes yields a substituted benzene – cyclization of 2-butyne forming hexamethylbenzene, for example. Benzene itself can be prepared by Reppe synthesis with the ligands on the nickel catalyst dictating whether production of benzene or cyclooctatetraene predominates. If an unsymmetric alkyne is used, a variety of products is formed; Rhodium-catalysed cyclotrimerization of phenylacetylene yields both the 1,2,4- and 1,3,5- isomers of triphenylbenzene along with substantial quantities of acyclic enyne products, though use of an alternative catalyst system can produce 97% selectivity for the 1,2,4-product. Antiaromatic cyclobutadiene-derivatives and non-aromatic cyclooctatetraene products are also observed side-products of cyclotrimerizations, and when a mixture of substituted acetylenes is used regioselectivity becomes especially problematic. Replacement of one acetylene by a nitrile allows formation of pyridine or one of its derivatives by Bönnemann cyclization.

Cyclisation carried out with a diyne and a separate alkyne affords greater control as well as high atom economy. Using commercially-available cyclopentadienylcobalt dicarbonyl, CpCo(CO)2, as catalyst, bis(trimethylsilyl)acetylene (BTMSA) will react with a diyne-1,2-disubstituted benzene to form an anthroquinone aromatic system as shown below: Benzyne, generated in situ from a bezene ring bearing ortho-distributed triflate and trimethylsilyl substituents, can be used to generate an aryne in place of an acetylene and combined with a suitable diyne. Such a benzene derivative reacts with 1,7-octadiyne in the presence of a suitable catalyst to generate a naphthalene system. This is an example of a hexadehydro Diels-Alder reaction.
If three alkyne moieties are tethered together, it is possible to create three rings in a single step without the problems with side products mentioned above. An example is seen in the synthesis of calomelanolactone using Wilkinson's catalyst to achieve an intramolecular cyclotrimerization.
Other cyclizations
The Bergman cyclization is conceptually similar, using a enediyne with (Z)-stereochemistry plus a hydrogen donor, catalysed by metal centre like ruthenium; (Z)-hex-3-en-1,5-diyne cyclizes to benzene after reduction of the intermediate diradical, for example. The enediyne moiety can be included within an existing ring, allowing access to a bicyclic system under mild conditions as a consequence of the ring strain in the reactant. Cyclodeca-3-en-1,5-diyne reacts with 1,3-cyclohexadiene to produce benzene and tetralin at 37 °C, the reaction being highly favorable owing to the formation of two new aromatic rings:
In the Wulff–Dötz reaction an alkyne, carbon monoxide and a chromium carbene complex are the reactants and the product is a chromium half-sandwich complex. The phenol ligand can be isolated by mild oxidation with ceric ammonium nitrate.

Diels–Alder reactions of alkynes with 2-pyrone or cyclopentadienone with expulsion of carbon dioxide or carbon monoxide, respectively, also form arene compounds. Tetracyclone reacts with diphenylacetylene to form hexaphenylbenzene after loss of carbon monoxide, for example. The Wagner-Jauregg reaction involves a double Diels-Alder addition of maleic anhydride to a 1,1-diarylethylene, resulting in an aryl-substituted naphthalene product. The reaction is unusual in that the aromaticity of the starting material is lost as the aryl-substituted styrene moiety is sufficiently activated to act as a dienophile, but the intermediate undergoes re-aromatization with sulfur.
In a Huisgen cycloaddition, an azide is combined with an alkyne to generate a triazole, and is a specific example of a 1,3-dipolar cycloaddition to a form a heterocyclic five-membered aromatic rings with regio- and stereoselectivity. American chemist Barry Sharpless has described this cycloaddition as "the cream of the crop" of click chemistry and "the premier example of a click reaction."
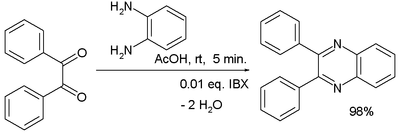
Condensation reactions are another route to the formation of heterocyclic aromatic systems. The Chichibabin pyridine synthesis involves condensation of acrolein (from a Knoevenagel reaction of acetaldehyde with formaldehyde) with acetaldehyde and ammonia, followed by catalysed oxidation of dihydropyridine to pyridine. Hantzsch's approach to pyridines dates from 1881 and involves condensation of a β-keto acid or ester with an aldehyde and a nitrogen donor (typicallyammonia or one of its salts. Knoevenagel modified this process for asymmetrically substituted pyridine derivatives. Quinoxaline systems can be formed by the condensation of 1,2-diketones with o-diaminobenzene, quinoxaline itself forming from glyoxal and o-phenylenediamine. Combining benzil with o-phenylenediamine in the presence of 2-iodobenzoic acid (IBX, catalyst) yields 2,3-diphenylquinoxaline in high yield.

Another effective means of generating heterocyclic systems is to effect ring-closure using the heteroatom. "Moderately aromatic" arsole derivatives can be formed by dimerising diphenylacetylene with lithium to form 1,4-dilithiotetraphenyl-1,3-butadiene. Phenylarsenous dichloride is added to close the ring to form pentaphenylarsole. The diiodo analogue of the lithium salt can be used in its place, and other pnictogen heterocycles can be produced by replacing the arsenic compound as appropriate.
From existing cyclic systems

Non aromatic rings can be aromatized to become aromatic by a variety of simple transformations. Dehydration allows the Semmler-Wolff transformation of 2-cyclohexenone oxime to aniline under acidic conditions. In applying redox processes, 2,3-dichloro-5,6-dicyano-1,4-benzoquinone (DDQ) and an acid catalyst has been used to synthesise a steroid with a phenanthrene core by oxidatation accompanied by a double methyl migration. In the process, DDQ is itself reduced into an aromatic hydroquinone product.

The 10 π-electron system cyclodecapentaene is non-aromatic as it is non-planar, but aromatic derivatives can be prepared. In the following reaction sequence, naphthalene is converted through a series of non-aromatic intermediates to 1,6-methanoannulene by Birch reduction, carbene addition, and then DDQ oxidation. Many other conversions of one aromatic system to another are known. One simple example is the industrial synthesis of pyrrole by exchanging the oxygen atom in furan for a nitrogen moiety. Ammonia and solid acid catalysts (like SiO2 and Al2O3) are required. Pyrrole can be converted to 3-chloropyridine by insertion of a carbene into the five-member ring. Dichlorocarbene adds to form a strained bicyclic cyclopropane system which then opens to form the six-member pyridine product, a transformation known as the Ciamician-Dennstedt rearrangement.
Aromatase is an enzyme which converts some enones with a six membered ring to aromatic ring - specifically testosterone to estradiol and androstenedione to estrone. Each of these aromatizations involves the oxidation of the C-19 methyl group to formic acid to allow for the formation of the aromatic system, conversions which are necessary parts of estrogen tumorogenesis in the development of breast cancer and ovarian cancer in postmenopausal women and gynecomastia in men. Aromatase inhibitors like exemestane (which forms a permanent and deactivating bond with the aromatase enzyme) and anastrozole and letrozole (which compete for the enzyme) have been shown to be more effective than anti-estrogen medications such as tamoxifen likely because they prevent the formation of estradiol.
Ionic systems

Aromaticity can be found in individual ions which satisfy Hückel's rule and they can be accessed by simple redox or acid-base reactions. A Hückel n = 0 cation can be prepared from cyclopropene systems, an example being the bromide salt of the triphenylcycloprenium cation. Ionic Hückel n = 1 systems are known both for anions (cyclopentadienyl) and cations (tropylium). Tropylium, C
7H
7, was first reported in 1881 though its identity was not recognized for over 60 years. Tropylium appears regularly in mass spectrometry, given a signal at m/z = 91. Compounds containing the benzyl moiety produce the molecular fragment PhCH
2 which isomeizes to the stable tropylium cation. Cycloheptatriene (non-aromatic) undergoes a redox reaction with bromine produces tropylium with the release of hydrogen bromide. It can also be oxidised with chromic acid and a ring-contracting rearrangement occurs in which benzaldehyde is produced:
- C
7H
8 + Br
2 → C
7H
7 + Br
+ HBr
- C
7H
7 + HCrO
4 → C
6H
5CHO + CrO
2 + H
2O

Cyclopentadiene is more acidic than a typical hydrocarbon (pKa ~ 15-16) and so can be readily deprotonated to its aromatic conjugate base, an approach used to form compounds like thallium cyclopentadienide, an example of an organometallic polymer. A direct redox reaction of sodium metal with cyclopentadiene yields sodium cyclopentadienide, though a convenient base like sodium hydride can also be used. Compounds like these can be used to prepare metallocene compounds such as ferrocene where two cyclopentadienyl ligands coordinated to a metal centre. Freshly-cracked cyclopentadiene will react with iron(II) chloride and a base to effect deprotonation to produce ferrocene in good yield. The aromaticity of the product can be seen as it undergoes Friedel-Crafts acylation with acetic anhydride.


Cyclooctatetraene has been shown to be non-aromatic and to possess a bent "tub-shaped" conformation with alternating double and single bonds. By contrast, its dianionC
8H
8 is both planar and aromatic and has a Hückel electron count of 10 It is formed readily by direct reaction with potassium metal and uranocene, an analogue of ferrocene, has been prepared from dipotassium cyclooctatetraenide and uranium tetrachloride in tetrahydrofuran at 0 °C:
Isomerization

An isomerization reaction involves the rearrangement of atoms within a single molecule to one of its isomers. Some isomerization processes occur spontaneously resulting in an equilibrium between the isomers, and the position of the system generally favors an aromatic product if the process is itself an aromatization. Amongst keto-enol tautomers, the keto form is generally favoured on thermodynamic grounds, but in the case of cyclohexa-2,4-dienone its aromatic isomer phenol is very strongly favoured. The equilibrium constant for this system has been determined to be 10, meaning that in a bottle of phenol there are 10 trillion molecules in the aromatic enol form for every molecule in the keto form. Isomerization reactions are temperature dependent, for example melting 1,4-naphthalenediol at 200 °C produces a 2:1 mixture with its keto form, 1,4-dioxotetralin. The conversion to the 1,4-dioxotetralin tautomer can be driven to completion when bound in a chromium tricarbonyl piano stool complex.

A non-tautomeric example of isomerization can be seen in the azulene–napthalene system, a thermal rearrangement in which each of the compounds is stable at room temperature; such interconversion aromatization reactions are rare. Carbon-13 isotope studies have shown scrambling of the α- and β- positions of naphthalene through an azulene intermediate at elevated temperatures though naphthalene has roughly double the aromatic stabilization of azulene. The synthesis of the azulene system is an example of a multi-step organic synthesis, in this case from cycloheptatriene, as shown below. Azulene is unusual in that it is a polar hydrocarbon – the dipole moment of azulene is 1.08 D compared with naphthalene's 0 D. This can be understood by recognizing that one of the important resonance contributors for azulene has the tropylium cation fused to the cyclopentadienyl anion; reactivity studies confirm the electrophilic and nucleophilic characteristics of these rings, respectively.
See also
References
- ^ Reppe, W.; Schlichting, O.; Klager, K.; Toepel, T. (1948). "Cyclisierende Polymerisation von Acetylen I Über Cyclooctatetraen". Justus Liebigs Annalen der Chemie (in German). 560: 1–92. doi:10.1002/jlac.19485600102.
- Weber, S. R.; Brintzinger, H. H. (1977). "Reactions of Bis(hexamethylbenzene)iron(0) with Carbon Monoxide and with Unsaturated Hydrocarbons". Journal of Organometallic Chemistry. 127 (1): 45–54. doi:10.1016/S0022-328X(00)84196-0.
- Ardizzoia, G. A.; Brenna, S.; Cenini, S.; LaMonica, G.; Masciocchi, N.; Maspero, A. (2003). "Oligomerization and Polymerization of Alkynes Catalyzed by Rhodium(I) Pyrazolate Complexes". Journal of Molecular Catalysis A: Chemical. 204–205: 333–340. doi:10.1016/S1381-1169(03)00315-7.
- Hilt, G.; Vogler, T.; Hess, W.; Galbiati, F. (2005). "A Simple Cobalt Catalyst System for the Efficient and Regioselective Cyclotrimerisation of Alkynes". Chemical Communications. 2005 (11): 1474–1475. doi:10.1039/b417832g.
- Behr, A. (2008). Angewandte homogene Katalyse (in German). Wiley-VCH. ISBN 9783527316663.
- Hillard, R. L.; Vollhardt, K. P. C. (1977). "Substituted Benzocyclobutenes, Indans, and Tetralins via Cobalt-Catalyzed Cooligomerization of α,ω-diynes with Substituted Acetylenes. Formation and Synthetic Utility of Trimethylsilylated Benzocycloalkenes". Journal of the American Chemical Society. 99 (12): 4058–4069. doi:10.1021/ja00454a026.
- Hsieh, J.-C.; Cheng, C.-H. (2005). "Nickel-Catalyzed Cocyclotrimerization of Arynes with Diynes; A Novel Method for Synthesis of Naphthalene Derivatives". Chemical Communications. 2005 (19): 2459–2461. doi:10.1039/b415691a.
- Neeson, S. J.; Stevenson, P. J. (1988). "Rhodium Catalysed Cycloadditions – An Efficient Regiospecific Route to Calomelanolactone". Tetrahedron Letters. 29 (7): 813–814. doi:10.1016/S0040-4039(00)80217-8.
- Odedra, A.; Wu, C.-J.; Pratap, T. B.; Huang, C.-W.; Ran, Y.-F.; Liu, R.-S. (2005). "Ruthenium-Catalyzed Aromatization of Enediynes via Highly Regioselective Nucleophilic Additions on a π-Alkyne Functionality. A Useful Method for the Synthesis of Functionalized Benzene Derivatives". Journal of the American Chemical Society. 127 (10): 3406–3412. doi:10.1021/ja043047j.
- Mohamed, R. K.; Peterson, P. W.; Alabugin, I. V. (2013). "Concerted Reactions that Produce Diradicals and Zwitterions: Electronic, Steric, Conformational and Kinetic Control of Cycloaromatization Processes". Chemical Reviews. 113 (9): 7089–7129. doi:10.1021/cr4000682.
- Waters, M.; Wulff, W. D. (2008). "The Synthesis of Phenols and Quinones via Fischer Carbene Complexes". Organic Reactions. 70 (2): 121–623. doi:10.1002/0471264180.or070.02.
- Dötz, K. H. (1983). "Carbon-Carbon Bond Formation via Carbonyl-Carbene Complexes". Pure and Applied Chemistry. 55 (11). doi:10.1351/pac198355111689.
- Woodard, B. T.; Posner, G. H. (1999). "Recent Advances in Diels-Alder Cycloadditions Using 2-Pyrones". In Harmata, M. (ed.). Advances in Cycloaddition. Vol. 5. JAI Press. pp. 47–83. doi:10.1002/chin.199935304. ISBN 9780762303465.
- Fieser, L. F. (1966). "Hexaphenylbenzene". Organic Syntheses. 46: 44; Collected Volumes, vol. 5, p. 604. doi:10.15227/orgsyn.046.0044
- Bergmann, F.; Szmuszkowicz, J.; Fawaz, G. (1947). "The Condensation of 1,1-Diarylethylenes with Maleic Anhydride". Journal of the American Chemical Society. 69 (7): 1773–1777. doi:10.1021/ja01199a055.
- Huisgen, Rolf (1963). "1.3-Dipolare Cycloadditionen Rückschau und Ausblick". Angewandte Chemie (in German). 75: 604–637. doi:10.1002/ange.19630751304.
- Kolb, H. C.; Finn, M. G.; Sharpless, K. B. (2001). "Click Chemistry: Diverse Chemical Function from a Few Good Reactions". Angewandte Chemie International Edition. 40 (11): 2004–2021. doi:10.1002/1521-3773(20010601)40:11<2004::AID-ANIE2004>3.0.CO;2-5.
- Kolb, H. C.; Sharpless, B. K. (2003). "The Growing Impact of Click Chemistry on Drug Discovery". Drug Discovery Today. 8 (24): 1128–1137. doi:10.1016/S1359-6446(03)02933-7.
- Frank, R.L.; Seven, R. P. (1949). "Pyridines. IV. A Study of the Chichibabin Synthesis". Journal of the American Chemical Society. 71 (8): 2629–2635. doi:10.1021/ja01176a008.
- Shimizu, S.; Watanabe, N.; Kataoka, T.; Shoji, T.; Abe, N.; Morishita, S.; Ichimura, H. (2005). "Pyridine and Pyridine Derivatives". Ullmann's Encyclopedia of Industrial Chemistry. Wiley-VCH. doi:10.1002/14356007.a22_399.
- Hantzsch, A. (1881). "Condensationsprodukte aus Aldehydammoniak und ketonartigen Verbindungen". Berichte der Deutschen Chemischen Gesellschaft (in German). 14 (2): 1637–1638. doi:10.1002/cber.18810140214.
- Knoevenagel, E.; Fries, A. (1898). "Synthesen in der Pyridinreihe. Ueber eine Erweiterung der Hantzsch'schen Dihydropyridinsynthese". Berichte der Deutschen Chemischen Gesellschaft (in German). 31 (1): 761–767. doi:10.1002/cber.189803101157.
- Jones, R. G.; McLaughlin, K. C. (1950). "2,3-Pyrazinedicarboxylic Acid". Organic Syntheses. 30: 86. doi:10.15227/orgsyn.030.0086.
- Heravi, M. M.; Bakhtiari, K.; Tehrani, M. H.; Javadi, N. M.; Oskooie, H. A. (2006). "Facile Synthesis of Quinoxaline Derivatives Using o-Iodoxybenzoic Acid (IBX) at Room Temperature". Arkivoc. 2006 (16): 16–22. doi:10.3998/ark.5550190.0007.g02.
- Johansson, M. P.; Juselius, J. (2005). "Arsole Aromaticity Revisited". Letters in Organic Chemistry. 2: 469–474. doi:10.2174/1570178054405968.
Using quantum chemical methodology, we reinvestigate the aromaticity of the much debated arsole, using the newly developed gauge-including magnetically induced currents (GIMIC) method. GIMIC provides a quantitative measure of the induced ring current strength, showing arsole to be moderately aromatic.
- Braye, E. H.; Hübel, W.; Caplier, I. (1961). "New Unsaturated Heterocyclic Systems. I". Journal of the American Chemical Society. 83 (21): 4406–4413. doi:10.1021/ja01482a026.
- Horning, E. C.; Stromberg, V. L.; Lloyd, H. A. (1952). "Beckmann Rearrangements. An Investigation of Special Cases". Journal of the American Chemical Society. 74 (20): 5153–5155. doi:10.1021/ja01140a048.
- Brown, W.; Turner, A. B. (1971). "Applications of High-Potential Quinones. Part VII. The Synthesis of Steroidal Phenanthrenes by Double Methyl Migration". Journal of the Chemical Society C: Organic: 2566–2572. doi:10.1039/J39710002566.
- Vogel, E.; Klug, W.; Breuer, A. (1974). "1,6-Methanoannulene". Organic Synthesis. 54: 11. doi:10.15227/orgsyn.054.0011.
- Harreus, A. L. (2002). "Pyrrole". Ullmann's Encyclopedia of Industrial Chemistry. Wiley-VCH. doi:10.1002/14356007.a22_453.
- Skell, P. S.; Sandler, S. R. (1958). "Reactions of 1,1-Digalocyclopropanes with Electrophilic Reagents. Synthetic Route for Inserting a Carbon Atom Between the Atoms of a Double Bond". Journal of the American Chemical Society. 80 (8): 2024–2025. doi:10.1021/ja01541a070.
- ^ Avendaño, C.; Menéndez, J. C. (2008). "Aromatase Inhibitors". Medicinal Chemistry of Anticancer Drugs. Elsevier. pp. 65–73. doi:10.1016/B978-0-444-52824-7.00003-2. ISBN 9780080559629.
- Jasek, W., ed. (2007). Austria-Codex (in German) (62nd ed.). Vienna: Österreichischer Apothekerverlag. pp. 656–660. ISBN 9783852001814.
- Dinnendahl, V.; Fricke, U., eds. (2007). Arzneistoff-Profile (in German). Vol. 4 (21st ed.). Eschborn, Germany: Govi Pharmazeutischer Verlag. ISBN 9783774198463.
- Xu, R.; Breslow, R. (1997). "1,2,3-Triphenylcyclopropenium Bromide". Organic Syntheses. 74: 72. doi:10.15227/orgsyn.074.0072.
- Merling, G. (1891). "Ueber Tropin". Berichte der Deutschen Chemischen Gesellschaft (in German). 24 (2): 3108–3126. doi:10.1002/cber.189102402151.
- Doering, W. von E.; Knox, L. H. (1954). "The Cycloheptatrienylium (Tropylium) Ion". Journal of the American Chemical Society. 76 (12): 3203–3206. doi:10.1021/ja01641a027.
- Balaban, A. T.; Oniciu, D. C.; Katritzky, A. R. (2004). "Aromaticity as a Cornerstone of Heterocyclic Chemistry". Chemical Reviews. 104 (5): 2777–2812. doi:10.1021/cr0306790.
- Agarwai, O. P. (2009). Reactions and Reagents (46th ed.). Krishna Prakashan Media. pp. 614–615. ISBN 9788187224655.
- Nielson, A. J.; Rickard, C. E. F.; Smith, J. M. (1986). "Cyclopentadienylthallium (Thallium Cyclopentadienide)". Inorganic Syntheses. 24: 97–99. doi:10.1002/9780470132555.ch31.
- Olbrich, F.; Behrens, U. (1997). "Crystal Structure of Catena-Cyclopentadienyl Thallium, ". Zeitschrift für Kristallographie – New Crystal Structures. 212 (1): 47–47. doi:10.1524/ncrs.1997.212.1.47.
- Cotton, F. A.; Wilkinson, G. (1999). Advanced Inorganic Chemistry (6th ed.). John Wiley and Sons. ISBN 9780471199571.
- Girolami, G. S.; Rauchfuss, T. B.; Angelici, R. J. (1999). Synthesis and Technique in Inorganic Chemistry. University Science Books. ISBN 0935702482.
- Wilkinson, G. (1956). "Ferrocene". 36: 31. doi:10.15227/orgsyn.036.0031.
{{cite journal}}
: Cite journal requires|journal=
(help) - Bozak, R. E. (1966). "Acetylation of Ferrocene: A Chromatography Experiment for Elementary Organic Laboratory". Journal of Chemical Education. 43 (2): 73. doi:10.1021/ed043p73.
- Johnson, A. W. (1947). "Organic Chemistry". Science Progress. 35 (139): 506-515.
- Kaufman, H. S.; Fankuchen, I.; Mark, H. (1948). "Structure of Cyclo-octatetraene". Nature. 161 (4083): 165. doi:10.1038/161165a0.
- Thomas, P. M.; Weber, A. (1978). "High Resolution Raman Spectroscopy of Gases with Laser Sources. XIII – The Pure Rotational Spectra of 1,3,5,7-Cyclooctatetraene and 1,5-Cyclooctadiene". Journal of Raman Spectroscopy. 7 (6): 353–357. doi:10.1002/jrs.1250070614.
- Katz, T. J. (1960). "The Cyclooctatetraenyl Dianion". Journal of the American Chemical Society. 82 (14): 3784–3785. doi:10.1021/ja01499a077.
- Streitwieser, A.; Mueller-Westerhoff, U. (1968). "Bis(cyclooctatetraenyl)uranium (Uranocene). A New Class of Sandwich Complexes that Utilize Atomic f Orbitals". Journal of the American Chemical Society. 90 (26): 7364–7364. doi:10.1021/ja01028a044.
- Clayden, J.; Greeves, N.; Warren, S.; Wothers, P. (2001). Organic Chemistry (1st ed.). Oxford University Press. p. 531. ISBN 9780198503460.
- Capponi, M.; Gut, I. G.; Hellrung, B.; Persy, G.; Wirz, J. (1999). "Ketonization Equilibria of Phenol in Aqueous Solution". Canadian Journal of Chemistry. 77 (5–6): 605–613. doi:10.1139/cjc-77-5-6-605.
- Kündig, E. P.; Garcia, A. E.; Lomberget, T.; Bernardinelli, G. (2005). "Rediscovery, Isolation, and Asymmetric Reduction of 1,2,3,4-Tetrahydronaphthalene-1,4-dione and Studies of its Complex". Angewandte Chemie International Edition. 45 (1): 98–101. doi:10.1002/anie.200502588.
- Scott, L. T. (1982). "Thermal Rearrangements of Aromatic Compounds". Accounts of Chemical Research. 15 (2): 52–58. doi:10.1021/ar00074a004.
- Scott, L. T.; Kirms, M. A. (1981). "Azulene Thermal Rearrangements. C–Labeling Studies of Automerization and Isomerization to Naphthalene". Journal of the American Chemical Society. 103 (19): 5875–5879. doi:10.1021/ja00409a042.
- Scott, L. T.; Agopian, G. K. (1977). "Automerization of Naphthalene". Journal of the American Chemical Society. 99 (13): 4506–4507. doi:10.1021/ja00455a053.
- Carret, S.; Blanc, A.; Coquerel, Y.; Berthod, M.; Greene, A. E.; Deprés, J.-P. (2005). "Approach to the Blues: A Highly Flexible Route to the Azulenes". Angewandte Chemie International Edition. 44 (32): 5130–5133. doi:10.1002/anie.200501276.
- Anderson, A. G.; Stecker, B. M. (1959). "Azulene. VIII. A Study of the Visible Absorption Spectra and Dipole Moments of Some 1- and 1,3-Substituted Azulenes". Journal of the American Chemical Society. 81 (18): 4941–4946. doi:10.1021/ja01527a046.
![]() | This chemical reaction article is a stub. You can help Misplaced Pages by expanding it. |