![]() | It has been suggested that this article be merged into Fatigue (material). (Discuss) Proposed since August 2024. |
![]() | This article has multiple issues. Please help improve it or discuss these issues on the talk page. (Learn how and when to remove these messages)
|
Static fatigue describes how prolonged and constant cyclic stress weakens a material until it breaks apart, which is called failure. Static fatigue is sometimes called "delayed fracture". The damage occurs at a lower stress level than the stress level needed to create a normal tensile fracture. Static fatigue can involve plastic deformation or crack growth. For example, repeated stress can create small cracks that grow and eventually break apart plastic, glass, or ceramic materials. The material reaches failure faster by increasing cyclic stress. Static fatigue varies with material type and environmental factors, such as moisture presence and temperature.
Applications
Static fatigue tests can estimate a material’s lifetime and hardness to different environments. However, measuring a static fatigue limit can take a long time, and it is hard to measure a material’s true static fatigue limit with full certainty.
Typical occurrence
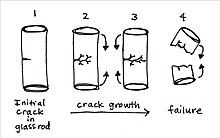
Stress corrosion cracking
Main article: Stress corrosion crackingStress corrosion cracking (SCC) happens when a stressed material is in a corrosive (chemically destructive) environment. One example of SSC embrittlement is when moisture increases static fatigue effects in glass. SCC is also seen in hydrogen embrittlement, embrittlement of some polymers, and more.
Plastic Deformation (Plastic Flow)
Plastic deformation happens when stresses flatten, bend, or twist a material until it cannot return to its original shape. This can create cracks in the material and decrease its lifetime ultimately leading to the deformation of plastic.
Examples of Static Fatigue and Stresses on Materials
Plastic pipes under water or other fluids experience hydrodynamic forces that can result in fatigue. The pipes reach failure sooner as temperatures and exposure to aggressive substances increase. For static fatigue tests, rotating machines apply weight on the material under study causing it to bend in different directions, which weakens the material overtime.
References
- In Materials, Woodhead Publishing (2010). Guedes, Rui Miranda (ed.). Creep and Fatigue in Polymer Matrix Composites (1st ed.). Woodhead Publishing. ISBN 9780081014585.
- ^ Pelleg, Josh (2021). Cyclic Deformation in Oxides, Carbides, and Nitrides. Vol. 22. Springer. pp. 495–511. doi:10.1007/978-3-030-86118-6_15. ISBN 978-3-030-86118-6. S2CID 244421914.
- ^ Wang, G.S.; Blom, A.F. "Effect of Large Local Plastic Flow on the Fatigue Life of Metallic Materials". Aeronautics Division – via The Swedish Defence Research Agency.
- Courtney, Thomas H. (2005-12-16). Mechanical Behavior of Materials: Second Edition. Waveland Press. ISBN 9781478608387.
- Furmanski, J.; Rimnac, C.M. (2011). "Crack Propagation Resistance Is Similar Under Static and Cyclic Loading in Crosslinked UHMWPE: A Pilot Study". Clinical Orthopaedics and Related Research. 469 (8): 2302–2307. doi:10.1007/s11999-010-1712-y. PMC 3126950. PMID 21128033.
- Crawford, Roy. J. (1998). Plastics Engineering (Chapter 1 - General Properties of Plastics) (1 ed.). Matthew Deans. pp. 1–40. ISBN 9780081007099.
- Grutzik, S.J.; Strong, K.T.; Rimsza, J.M. (December 2022). "Kinetic model for prediction of subcritical crack growth, crack tip relaxation, and static fatigue threshold in silicate glass". Journal of Non-Crystalline Solids: X. 16 (100134): 100134. Bibcode:2022JNCSX..1600134G. doi:10.1016/j.nocx.2022.100134. S2CID 254308009.
- Ruys, Andrew (2019). Processing, structure, and properties of alumina ceramics. Woodhead Publishing. pp. 71–121. doi:10.1016/C2017-0-01189-8. ISBN 978-0-08-102442-3.
- Laughton, M.J.; Warne, D.J.; Tricker, R. (2003). Optical Fibres in Power Systems (16 ed.). Newnes. pp. 37–1, 37-3 – 37-17. doi:10.1016/B978-075064637-6/50037-X. ISBN 978-0-7506-4637-6.
- Kingery, W.D. (1976). Introduction to ceramics. New York: Wiley. ISBN 978-0471478607.
- Ebel, A.; Caty, O.; Rebillat, F. (2022). "Effect of temperature on static fatigue behavior of self-healing CMC in humid air". Composites Part A: Applied Science and Manufacturing. 157: 106899. doi:10.1016/j.compositesa.2022.106899. S2CID 247148726.
- ^ Wilkins, B.J.; Dutton, R. (March 1976). "Static Fatigue Limit with Particular Reference to Glass". Journal of the American Ceramic Society. 59 (3–4): 108–112. doi:10.1111/j.1151-2916.1976.tb09442.x.
- Kelly, A.; Zweben, C.; Sims, G.D.; Broughton, W.R. (2000). Comprehensive Composite Materials (2.05 - Glass Fiber Reinforced Plastics—Properties). Vol. 2. Pergamon. pp. 151–197. doi:10.1016/B0-08-042993-9/00181-9. ISBN 978-0-08-042993-9.
- Raja, V.S.; Tetsuo, Shoji (2011). Stress Corrosion Cracking. Woodhead Publishing. pp. 245–272. doi:10.1533/9780857093769.3.245. ISBN 978-1-84569-673-3.
- Wiederhorn, S. M.; Bolz, L. H. (1970-10-01). "Stress Corrosion and Static Fatigue of Glass". Journal of the American Ceramic Society. 53 (10): 543–548. doi:10.1111/j.1151-2916.1970.tb15962.x. ISSN 1551-2916.
- Lou than, M.R. "Hydrogen Embrittlement - Office of Scientific and Technical Information" (PDF).
- Murakami, Y.; Kanezaki, T.; Matsuoka, S. (2008). "Hydrogen Embrittlement Mechanism in Fatigue of Austenitic Stainless Steels". Metallurgical and Materials Transactions A. 39 (6): 1327–1339. Bibcode:2008MMTA...39.1327M. doi:10.1007/s11661-008-9506-5. S2CID 137234625.
- Brown, Norman; Parrish, Mark F. (1974). Bishay, Adli (ed.). Recent Advances in Science and Technology of Materials. Springer US. pp. 1–13. doi:10.1007/978-1-4613-4538-1_1. ISBN 9781461345404.
- Pfeifer, Michael (2009). "Chapter 6 - Degradation and Reliability of Materials". Materials Enabled Designs. Butterworth Heinemann. pp. 161–187. doi:10.1016/B978-0-7506-8287-9.00006-9. ISBN 978-0-7506-8287-9 – via Science Direct.
- ^ Farshad, Mehdi (2006-01-01), Farshad, Mehdi (ed.), "7 - Fatigue, corrosion, and wear", Plastic Pipe Systems, Oxford: Elsevier Science, pp. 153–165, ISBN 978-1-85617-496-1, retrieved 2023-04-15
- Goodman, Soderberg (2022), "Fatigue", MET 301: Design for Cyclic Loading (PDF), New Jersey Institute of Technology, p. 1