Revision as of 12:59, 8 August 2015 view sourceSeppi333 (talk | contribs)Autopatrolled, Extended confirmed users, Page movers, New page reviewers, Pending changes reviewers, Template editors35,345 edits AU+NZ← Previous edit | Revision as of 18:02, 8 August 2015 view source Klermodalwonfeyz (talk | contribs)Extended confirmed users3,766 editsNo edit summaryTag: Visual editNext edit → | ||
Line 80: | Line 80: | ||
READ THIS BEFORE EDITING: every medical statement in the lead has a reference in the body of the article. Please do not delete contested lead content without either looking for the statement's ref in the body of the article and/or asking about it on the talk page first. | READ THIS BEFORE EDITING: every medical statement in the lead has a reference in the body of the article. Please do not delete contested lead content without either looking for the statement's ref in the body of the article and/or asking about it on the talk page first. | ||
-->'''Methamphetamine'''{{#tag:ref|Synonyms and alternate spellings include: metamfetamine (]), ''N''-methylamphetamine, desoxyephedrine, Syndrox, and Desoxyn.<ref name="EMCDDA profile">{{cite web |url=http://www.emcdda.europa.eu/publications/drug-profiles/methamphetamine |title=Methamphetamine |date=16 August 2010 |work=Drug profiles |publisher=] (EMCDDA) |accessdate=1 September 2011}}</ref><ref name="DB ID">{{cite encyclopedia | title=Methamphetamine | section-url=http://www.drugbank.ca/drugs/DB01577#identification | work=DrugBank | publisher= University of Alberta | accessdate=31 December 2013 | date=8 February 2013 | section=Identification }}</ref> Common slang terms for methamphetamine include: speed, meth, crystal, crystal meth, glass, shards, ice, and tic<ref>{{cite web|title=Meth Slang Names|url=http://www.methhelponline.com/meth-slang.htm|work=MethhelpOnline|accessdate=1 January 2014}}</ref> and, in New Zealand, "P".<ref>http://www.police.govt.nz/advice/drugs-and-alcohol/methamphetamine-and-law</ref>| group = "note" }} ({{IPAc-en|pron|ˌ|m|ɛ|θ|æ|m|ˈ|f|ɛ|t|əm|iː|n}}; contracted from {{nowrap|]-]}}) is a strong ] (CNS) ] that is used as a ] and |
-->'''Methamphetamine'''{{#tag:ref|Synonyms and alternate spellings include: metamfetamine (]), ''N''-methylamphetamine, desoxyephedrine, Syndrox, and Desoxyn.<ref name="EMCDDA profile">{{cite web |url=http://www.emcdda.europa.eu/publications/drug-profiles/methamphetamine |title=Methamphetamine |date=16 August 2010 |work=Drug profiles |publisher=] (EMCDDA) |accessdate=1 September 2011}}</ref><ref name="DB ID">{{cite encyclopedia | title=Methamphetamine | section-url=http://www.drugbank.ca/drugs/DB01577#identification | work=DrugBank | publisher= University of Alberta | accessdate=31 December 2013 | date=8 February 2013 | section=Identification }}</ref> Common slang terms for methamphetamine include: speed, meth, crystal, crystal meth, glass, shards, ice, and tic<ref>{{cite web|title=Meth Slang Names|url=http://www.methhelponline.com/meth-slang.htm|work=MethhelpOnline|accessdate=1 January 2014}}</ref> and, in New Zealand, "P".<ref>http://www.police.govt.nz/advice/drugs-and-alcohol/methamphetamine-and-law</ref>| group = "note" }} ({{IPAc-en|pron|ˌ|m|ɛ|θ|æ|m|ˈ|f|ɛ|t|əm|iː|n}}; contracted from {{nowrap|]-]}}) is a strong ] (CNS) ] that is mainly used as a ]. ''Methamphetamine hydrochloride'' is approved by the ] (USFDA) under the trade name ''Desoxyn'' for ] and ] in children and adults, and also prescribed ] for ] and ]. It is rarely prescribed due to claims of toxicity. | ||
Methamphetamine was discovered in 1893 and exists as two ] ]s: dextromethamphetamine and ].{{#tag:ref|Enantiomers are molecules that are ''mirror images'' of one another; they are structurally identical, but of the opposite orientation.|group = "note"}} ''Methamphetamine'' properly refers to a specific chemical, the ] ],<!--As defined, the IUPAC name and INN refer to the racemate--> which is an equal parts mixture of levomethamphetamine and dextromethamphetamine in their pure amine forms. Dextromethamphetamine is a stronger CNS stimulant than levomethamphetamine; however, both are ], ], and produce the same toxicity symptoms when used at high doses. | |||
Methamphetamine and ] dextromethamphetamine are illicitly trafficked and sold throughout the world due to their potential for recreational use as an ] and ]; the highest prevalence of illegal methamphetamine use occurs in parts of Asia and Oceania and in the United States, where racemic methamphetamine and pure dextromethamphetamine are classified as ] controlled substances. In contrast, enantiopure levomethamphetamine is an ] which is marketed as a ] in the United States.<ref name="Vicks" group="note" /> Internationally, the production, distribution, sale, and possession of methamphetamine is restricted or illegal in many other countries due to its placement in schedule II of the ] treaty. While pure dextromethamphetamine is a more potent drug than racemic methamphetamine, the racemic form is sometimes produced and sold instead of dextromethamphetamine due to the relative ease of ] by certain methods and the limited availability of associated ]. | Methamphetamine and ] dextromethamphetamine are illicitly trafficked and sold throughout the world due to their potential for recreational use as an ] and ]; the highest prevalence of illegal methamphetamine use occurs in parts of Asia and Oceania and in the United States, where racemic methamphetamine and pure dextromethamphetamine are classified as ] controlled substances. In contrast, enantiopure levomethamphetamine is an ] which is marketed as a ] in the United States.<ref name="Vicks" group="note" /> Internationally, the production, distribution, sale, and possession of methamphetamine is restricted or illegal in many other countries due to its placement in schedule II of the ] treaty. While pure dextromethamphetamine is a more potent drug than racemic methamphetamine, the racemic form is sometimes produced and sold instead of dextromethamphetamine due to the relative ease of ] by certain methods and the limited availability of associated ]. |
Revision as of 18:02, 8 August 2015
This article is about the free base and salts of methamphetamine. "Meth" redirects here. For other uses, see Meth (disambiguation)Pharmaceutical compound
![]() | |
![]() | |
Clinical data | |
---|---|
Trade names | Desoxyn |
Other names | N-methylamphetamine, desoxyephedrine |
AHFS/Drugs.com | Monograph |
License data | |
Dependence liability | Physical: none Psychological: high |
Addiction liability | Very high |
Routes of administration | Medical: oral Recreational: oral, intravenous, insufflation, inhalation, suppository |
ATC code | |
Legal status | |
Legal status |
|
Pharmacokinetic data | |
Bioavailability | Oral: Varies widely Rectal: 99% IV: 100% |
Protein binding | Varies widely |
Metabolism | CYP2D6, DBH, FMO3, XM-ligase, and ACGNAT |
Elimination half-life | 9–12 hours |
Excretion | Renal |
Identifiers | |
IUPAC name
| |
CAS Number | |
PubChem CID | |
IUPHAR/BPS | |
DrugBank | |
ChemSpider | |
UNII | |
KEGG | |
ChEBI | |
ChEMBL | |
CompTox Dashboard (EPA) | |
ECHA InfoCard | 100.007.882 ![]() |
Chemical and physical data | |
Formula | C10H15N |
Molar mass | 149.237 g·mol |
3D model (JSmol) | |
Melting point | 3 °C (37 °F) |
Boiling point | 212 °C (414 °F) at 760 MM HG |
SMILES
| |
InChI
| |
(verify) |
Methamphetamine ( /ˌmɛθæmˈfɛtəmiːn/; contracted from N-methyl-alpha-methylphenethylamine) is a strong central nervous system (CNS) stimulant that is mainly used as a recreational drug. Methamphetamine hydrochloride is approved by the United States Food and Drug Administration (USFDA) under the trade name Desoxyn for attention deficit hyperactivity disorder and obesity in children and adults, and also prescribed off label for narcolepsy and idiopathic hypersomnia. It is rarely prescribed due to claims of toxicity.
Methamphetamine was discovered in 1893 and exists as two dextrorotary and levorotary enantiomers: dextromethamphetamine and levomethamphetamine. Methamphetamine properly refers to a specific chemical, the racemic free base, which is an equal parts mixture of levomethamphetamine and dextromethamphetamine in their pure amine forms. Dextromethamphetamine is a stronger CNS stimulant than levomethamphetamine; however, both are neurotoxic, addictive, and produce the same toxicity symptoms when used at high doses.
Methamphetamine and enantiopure dextromethamphetamine are illicitly trafficked and sold throughout the world due to their potential for recreational use as an aphrodisiac and euphoriant; the highest prevalence of illegal methamphetamine use occurs in parts of Asia and Oceania and in the United States, where racemic methamphetamine and pure dextromethamphetamine are classified as schedule II controlled substances. In contrast, enantiopure levomethamphetamine is an over-the-counter drug which is marketed as a nasal decongestant in the United States. Internationally, the production, distribution, sale, and possession of methamphetamine is restricted or illegal in many other countries due to its placement in schedule II of the United Nations Convention on Psychotropic Substances treaty. While pure dextromethamphetamine is a more potent drug than racemic methamphetamine, the racemic form is sometimes produced and sold instead of dextromethamphetamine due to the relative ease of its synthesis by certain methods and the limited availability of associated chemical precursors.
In low doses, methamphetamine can cause an elevated mood and increase alertness, concentration, and energy in fatigued individuals. At higher doses, it can induce psychosis, rhabdomyolysis and cerebral hemorrhage. Recreationally, methamphetamine's ability to increase energy has been reported to lift mood and increase sexual desire to an extent of users ability to engage in sexual activity continuously for several days. It is of the phenethylamine and amphetamine classes. Methamphetamine is known to have a high potential for recreational misuse and addiction. Heavy recreational use of methamphetamine may result in psychosis or lead to post-acute-withdrawal syndrome, a withdrawal syndrome that can persist for months beyond the typical withdrawal period. Unlike amphetamine, methamphetamine is neurotoxic to humans, damaging both dopamine and serotonin neurons in the CNS. Contrary to the long-term use of amphetamine, there is evidence that methamphetamine causes brain damage from long-term use in humans; this damage includes adverse changes in brain structure and function, such as reductions in grey matter volume in several brain regions and adverse changes in markers of metabolic integrity.
Methamphetamine belongs to the substituted phenethylamine and substituted amphetamine chemical classes. It is related to the other dimethylphenethylamines as a positional isomer of these compounds, which share the common chemical formula: Template:Chemical formula.
Uses
Medical
In the United States, methamphetamine hydrochloride, under the trade name Desoxyn, has been approved by the FDA for treating severe ADHD and exogenous obesity (obesity originating from factors outside the patient's control) in both adults and children; however, the FDA also indicates that the limited therapeutic usefulness of methamphetamine should be weighed against the inherent risks associated with its use. Methamphetamine is sometimes prescribed off label for narcolepsy and idiopathic hypersomnia. In the United States, methamphetamine's levorotary form is available in some over-the-counter nasal decongestant products, such as Vicks VapoInhaler and its generic equivalents.
As methamphetamine is associated with a high potential for misuse, the drug is regulated under the Controlled Substances Act and is listed under schedule II in the United States. Methamphetamine hydrochloride dispensed in the United States is required to include the following boxed warning:
Methamphetamine has a high potential for abuse. It should thus be tried only in weight reduction programs for patients in whom alternative therapy has been ineffective. Administration of methamphetamine for prolonged periods of time in obesity may lead to drug dependence and must be avoided. Particular attention should be paid to the possibility of subjects obtaining methamphetamine for non-therapeutic use or distribution to others, and the drug should be prescribed or dispensed sparingly. Misuse of methamphetamine may cause sudden death and serious cardiovascular adverse effects.
Recreational
See also: Party and play and the Recreational routes of methamphetamine administrationMethamphetamine is often used recreationally for its effects as a potent euphoriant and stimulant as well as aphrodisiac qualities. According to a National Geographic TV documentary on methamphetamine, "an entire subculture known as party and play is based around methamphetamine use". Members of this San Francisco sub-culture, which consists almost entirely of gay male methamphetamine users, will typically meet up through internet dating sites and have sex. Due to its strong stimulant and aphrodisiac effects and inhibitory effect on ejaculation, with repeated use, these sexual encounters will sometimes occur continually for several days. The crash following the use of methamphetamine in this manner is very often severe, with marked hypersomnia (excessive daytime sleepiness).


Contraindications
Methamphetamine is contraindicated in individuals with a history of substance use disorder, heart disease, or severe agitation or anxiety, or in individuals currently experiencing arteriosclerosis, glaucoma, hyperthyroidism, or severe hypertension. The USFDA states that individuals who have experienced hypersensitivity reactions to other stimulants in the past or are currently taking monoamine oxidase inhibitors should not take methamphetamine. The USFDA also advises individuals with bipolar disorder, depression, elevated blood pressure, liver or kidney problems, mania, psychosis, Raynaud's phenomenon, seizures, thyroid problems, tics, or Tourette syndrome to monitor their symptoms while taking methamphetamine. Due to the potential for stunted growth, the USFDA advises monitoring the height and weight of growing children and adolescents during treatment.
Side effects
Physical
The physical effects of methamphetamine can include loss of appetite, hyperactivity, dilated pupils, flushed skin, excessive sweating, increased movement, dry mouth and teeth grinding (leading to "meth mouth"), headache, irregular heartbeat (usually as accelerated heartbeat or slowed heartbeat), rapid breathing, high blood pressure, low blood pressure, high body temperature, diarrhea, constipation, blurred vision, dizziness, twitching, numbness, tremors, dry skin, acne, and pale appearance. Methamphetamine that is present in a mother's bloodstream can pass through the placenta to a fetus and is or be secreted into breast milk. Infants born to methamphetamine-abusing mothers were found to have a significantly smaller gestational age-adjusted head circumference and birth weight measurements. Methamphetamine exposure was also associated with neonatal withdrawal symptoms of agitation, vomiting and fast breathing. This withdrawal syndrome is relatively mild and only requires medical intervention in approximately 4% of cases.
Meth mouth
Main article: Meth mouthMethamphetamine users and addicts may lose their teeth abnormally quickly, regardless of the route of administration, from a condition informally known as meth mouth. The condition is generally most severe in users who inject the drug, rather than those who smoke, ingest, or inhale it. According to the American Dental Association, meth mouth "is probably caused by a combination of drug-induced psychological and physiological changes resulting in xerostomia (dry mouth), extended periods of poor oral hygiene, frequent consumption of high-calorie, carbonated beverages and bruxism (teeth grinding and clenching)". Many researchers suggest that methamphetamine-induced tooth decay is due to users' lifestyles, as dry mouth is also a side effect of other stimulants, which are not known to cause serious tooth decay. They suggest that the side effect has been exaggerated and stylized to deter potential users and stereotype current users.
Sexually transmitted infection
Methamphetamine use was found to be related to higher frequencies of unprotected sexual intercourse in both HIV-positive and unknown casual partners, an association more pronounced in HIV-positive participants. These findings suggest that methamphetamine use and engagement in unprotected anal intercourse are co-occurring risk behaviors, behaviors that potentially heighten the risk of HIV transmission among gay and bisexual men. Methamphetamine use allows users of both sexes to engage in prolonged sexual activity, which may cause genital sores and abrasions as well as priapism in men. Methamphetamine may also cause sores and abrasions in the mouth via bruxism, increasing the risk of sexually transmitted infection.
Besides the sexual transmission of HIV, it may also be transmitted between users who share a common needle. The level of needle sharing among methamphetamine users is similar to that among other drug injection users.
Psychological
The psychological effects of methamphetamine can include euphoria, dysphoria, changes in libido, alertness, apprehension, concentration, decreased sense of fatigue, insomnia or wakefulness, self-confidence, sociability, irritability, restlessness, grandiosity and repetitive and obsessive behaviors. Methamphetamine use also has a high association with anxiety, depression, methamphetamine psychosis, suicide, and violent behaviors.
Neurotoxicity and neuroimmune response
![]() | This section needs expansion. You can help by making an edit requestadding to it . (August 2015) |

Unlike amphetamine, methamphetamine is directly neurotoxic to dopamine neurons. Moreover, methamphetamine neurotoxicity is associated with an increased risk of Parkinson's disease, an effect which partially arises through excessive cytosolic and synaptic autoxidation of dopamine. In addition to dopaminergic neurotoxicity, a review of evidence in humans also indicated that high-dose methamphetamine use can be neurotoxic to serotonin neurons. It has been demonstrated that a high core temperature is correlated with an increase in the neurotoxic effects of methamphetamine. As a result of methamphetamine-induced neurotoxicity to dopamine neurons, chronic use may also lead to post-acute withdrawal which persists months beyond the typical withdrawal period.
Overdose
A methamphetamine overdose may result in a wide range of symptoms. A moderate overdose of methamphetamine may induce symptoms such as: abnormal heart rhythm, confusion, difficult and/or painful urination, high or low blood pressure, high body temperature, over-active and/or over-responsive reflexes, muscle aches, severe agitation, rapid breathing, tremor, urinary hesitancy, and an inability to pass urine. An extremely large overdose may produce symptoms such as adrenergic storm, methamphetamine psychosis, substantially reduced or nil urine output, cardiogenic shock, brain bleed, circulatory collapse, dangerously high body temperature, pulmonary hypertension, kidney failure, rhabdomyolysis, serotonin syndrome, and a form of stereotypy ("tweaking"). A methamphetamine overdose will likely also result in mild brain damage due to dopaminergic and serotonergic neurotoxicity. Death from methamphetamine poisoning is typically preceded by convulsions and coma.
Psychosis
Abuse of methamphetamine can result in a stimulant psychosis which may present with a variety of symptoms (e.g. paranoia, hallucinations, delirium, delusions). A Cochrane Collaboration review on treatment for amphetamine, dextroamphetamine, and methamphetamine abuse-induced psychosis states that about 5–15% of users fail to recover completely. The same review asserts that, based upon at least one trial, antipsychotic medications effectively resolve the symptoms of acute amphetamine psychosis. Methamphetamine psychosis may also develop occasionally as a treatment-emergent side effect.
Emergency treatment
The USFDA states that acute methamphetamine intoxication is largely managed by treating the symptoms and treatments may initially include administration of activated charcoal and sedation. There is not enough evidence on hemodialysis or peritoneal dialysis in cases of methamphetamine intoxication to determine their usefulness. Forced acid diuresis (e.g., with vitamin C) will increase methamphetamine excretion but is not recommended as it may increase the risk of aggravating acidosis, or cause seizures or rhabdomyolysis. Hypertension presents a risk for intracranial hemorrhage and, if severe, is typically treated with intravenous phentolamine or nitroprusside. Blood pressure often drops gradually following sufficient sedation with a benzodiazepine and providing a calming environment. Chlorpromazine may be useful in decreasing the stimulant and CNS effects of a methamphetamine overdose. The use of a nonselective beta blocker may be required to control increased heart rate.
Addiction
Addiction and dependence glossary | |
---|---|
| |
Signaling cascade in the nucleus accumbens that results in psychostimulant addiction![]() ![]() |
Current models of addiction from chronic drug use involve alterations in gene expression in certain parts of the brain, particularly the nucleus accumbens. The most important transcription factors that produce these alterations are ΔFosB, cAMP response element binding protein (CREB), and nuclear factor kappa B (NFκB). ΔFosB plays a crucial role in the development of drug addictions, since its overexpression in D1-type medium spiny neurons in the nucleus accumbens is necessary and sufficient for most of the behavioral and neural adaptations that arise from addiction. Once ΔFosB is sufficiently overexpressed, it induces an addictive state that becomes increasingly more severe with further increases in ΔFosB expression. It has been implicated in addictions to alcohol, cannabinoids, cocaine, methylphenidate, nicotine, opioids, phencyclidine, propofol, and substituted amphetamines, among others.
ΔJunD, a transcription factor, and G9a, a histone methyltransferase enzyme, both directly oppose the induction of ΔFosB in the nucleus accumbens (i.e., they oppose increases in its expression). Sufficiently overexpressing ΔJunD in the nucleus accumbens with viral vectors can completely block many of the neural and behavioral alterations seen in chronic drug abuse (i.e., the alterations mediated by ΔFosB). ΔFosB also plays an important role in regulating behavioral responses to natural rewards, such as palatable food, sex, and exercise. Since both natural rewards and addictive drugs induce expression of ΔFosB (i.e., they cause the brain to produce more of it), chronic acquisition of these rewards can result in a similar pathological state of addiction. ΔFosB is the most significant factor involved in both amphetamine addiction and amphetamine-induced sex addictions, which are compulsive sexual behaviors that result from excessive sexual activity and amphetamine use. These sex addictions (i.e., drug-induced compulsive sexual behaviors) are associated with a dopamine dysregulation syndrome which occurs in some patients taking dopaminergic drugs, such as amphetamine or methamphetamine.
Treatment and management
Cognitive behavioral therapy is currently the most effective clinical treatment for psychostimulant addictions in general. As of May 2014, there is no effective pharmacotherapy for methamphetamine addiction. Methamphetamine addiction is largely mediated through increased activation of dopamine receptors and co-localized NMDA receptors in the nucleus accumbens. Magnesium ions inhibit NMDA receptors by blocking the receptor calcium channel.
Dependence and withdrawal
Tolerance is expected to develop with regular methamphetamine use and, when used recreationally, this tolerance develops rapidly. In dependent users, withdrawal symptoms are positively correlated with the level of drug tolerance. Depression from methamphetamine withdrawal lasts longer and is more severe than that of cocaine withdrawal.
According to the current Cochrane review on drug dependence and withdrawal in recreational users of methamphetamine, "when chronic heavy users abruptly discontinue use, many report a time-limited withdrawal syndrome that occurs within 24 hours of their last dose". Withdrawal symptoms in chronic, high-dose users are frequent, occurring in up to 87.6% of cases, and persist for three to four weeks with a marked "crash" phase occurring during the first week. Methamphetamine withdrawal symptoms can include anxiety, drug craving, dysphoric mood, fatigue, increased appetite, increased movement or decreased movement, lack of motivation, sleeplessness or sleepiness, and vivid or lucid dreams.
Protein For the silicon wafer box, see FOUP.
D-methamphetamine | |||||||||||||||||||||||||||||||||||||||||||||||||||
---|---|---|---|---|---|---|---|---|---|---|---|---|---|---|---|---|---|---|---|---|---|---|---|---|---|---|---|---|---|---|---|---|---|---|---|---|---|---|---|---|---|---|---|---|---|---|---|---|---|---|---|
![]() | |||||||||||||||||||||||||||||||||||||||||||||||||||
Identifiers | |||||||||||||||||||||||||||||||||||||||||||||||||||
Aliases | N-methylamphetamine pito-1342(S)-N,α-dimethylbenzeneethanaminepured-1-phenyl-2-methylaminopropanebaseicechalkS-methamphetaminetweakmethylamphetamine(+)-(S)-P-α-dimethylphenethylamineglassd-N-methylamphetamine(αS)-N,α-dimethylbenzeneethanamine(+)-(S)-N-alpha-dimethylphenethylaminewhizcrankd-phenylisopropylmethylaminemethyl-β-phenylisopropylaminemetamfetamined-desoxyephedrinecrystalspeedcrystal meth(alphaS)-N,alpha-dimethylbenzeneethanaminecrypto(S)-N,alpha-dimethylbenzeneethanaminefastdesoxyephedrinemethyl-beta-phenylisopropylamined-deoxyephedrinedextromethamphetaminemethamphetaminemethwaxd-meth | ||||||||||||||||||||||||||||||||||||||||||||||||||
External IDs | GeneCards: ; OMA:- orthologs | ||||||||||||||||||||||||||||||||||||||||||||||||||
| |||||||||||||||||||||||||||||||||||||||||||||||||||
Wikidata | |||||||||||||||||||||||||||||||||||||||||||||||||||
|
Protein fosB, also known as FosB and G0/G1 switch regulatory protein 3 (G0S3), is a protein that in humans is encoded by the FBJ murine osteosarcoma viral oncogene homolog B (FOSB) gene.
The FOS gene family consists of four members: FOS, FOSB, FOSL1, and FOSL2. These genes encode leucine zipper proteins that can dimerize with proteins of the JUN family (e.g., c-Jun, JunD), thereby forming the transcription factor complex AP-1. As such, the FOS proteins have been implicated as regulators of cell proliferation, differentiation, and transformation. FosB and its truncated splice variants, ΔFosB and further truncated Δ2ΔFosB, are all involved in osteosclerosis, although Δ2ΔFosB lacks a known transactivation domain, in turn preventing it from affecting transcription through the AP-1 complex.
The ΔFosB splice variant has been identified as playing a central, crucial role in the development and maintenance of addiction. ΔFosB overexpression (i.e., an abnormally and excessively high level of ΔFosB expression which produces a pronounced gene-related phenotype) triggers the development of addiction-related neuroplasticity throughout the reward system and produces a behavioral phenotype that is characteristic of an addiction. ΔFosB differs from the full length FosB and further truncated Δ2ΔFosB in its capacity to produce these effects, as only accumbal ΔFosB overexpression is associated with pathological responses to drugs.
DeltaFosB
DeltaFosB – more commonly written as ΔFosB – is a truncated splice variant of the FOSB gene. ΔFosB has been implicated as a critical factor in the development of virtually all forms of behavioral and drug addictions. In the brain's reward system, it is linked to changes in a number of other gene products, such as CREB and sirtuins. In the body, ΔFosB regulates the commitment of mesenchymal precursor cells to the adipocyte or osteoblast lineage.
In the nucleus accumbens, ΔFosB functions as a "sustained molecular switch" and "master control protein" in the development of an addiction. In other words, once "turned on" (sufficiently overexpressed) ΔFosB triggers a series of transcription events that ultimately produce an addictive state (i.e., compulsive reward-seeking involving a particular stimulus); this state is sustained for months after cessation of drug use due to the abnormal and exceptionally long half-life of ΔFosB isoforms. ΔFosB expression in D1-type nucleus accumbens medium spiny neurons directly and positively regulates drug self-administration and reward sensitization through positive reinforcement while decreasing sensitivity to aversion. Based upon the accumulated evidence, a medical review from late 2014 argued that accumbal ΔFosB expression can be used as an addiction biomarker and that the degree of accumbal ΔFosB induction by a drug is a metric for how addictive it is relative to others.
Chronic administration of anandamide, or N-arachidonylethanolamide (AEA), an endogenous cannabinoid, and additives such as sucralose, a noncaloric sweetener used in many food products of daily intake, are found to induce an overexpression of ΔFosB in the infralimbic cortex (Cx), nucleus accumbens (NAc) core, shell, and central nucleus of amygdala (Amy), that induce long-term changes in the reward system.
Role in addiction
Addiction and dependence glossary | |
---|---|
| |
Signaling cascade in the nucleus accumbens that results in psychostimulant addiction![]() ![]() |
Chronic addictive drug use causes alterations in gene expression in the mesocorticolimbic projection, which arise through transcriptional and epigenetic mechanisms. The most important transcription factors that produce these alterations are ΔFosB, cyclic adenosine monophosphate (cAMP) response element binding protein (CREB), and nuclear factor kappa B (NF-κB). ΔFosB is the most significant biomolecular mechanism in addiction because the overexpression of ΔFosB in the D1-type medium spiny neurons in the nucleus accumbens is necessary and sufficient for many of the neural adaptations and behavioral effects (e.g., expression-dependent increases in drug self-administration and reward sensitization) seen in drug addiction. ΔFosB overexpression has been implicated in addictions to alcohol, cannabinoids, cocaine, methylphenidate, nicotine, opioids, phencyclidine, propofol, and substituted amphetamines, among others. ΔJunD, a transcription factor, and G9a, a histone methyltransferase, both oppose the function of ΔFosB and inhibit increases in its expression. Increases in nucleus accumbens ΔJunD expression (via viral vector-mediated gene transfer) or G9a expression (via pharmacological means) reduces, or with a large increase can even block, many of the neural and behavioral alterations seen in chronic drug abuse (i.e., the alterations mediated by ΔFosB). Repression of c-Fos by ΔFosB, which consequently further induces expression of ΔFosB, forms a positive feedback loop that serves to indefinitely perpetuate the addictive state.
ΔFosB also plays an important role in regulating behavioral responses to natural rewards, such as palatable food, sex, and exercise. Natural rewards, similar to drugs of abuse, induce gene expression of ΔFosB in the nucleus accumbens, and chronic acquisition of these rewards can result in a similar pathological addictive state through ΔFosB overexpression. Consequently, ΔFosB is the key mechanism involved in addictions to natural rewards (i.e., behavioral addictions) as well; in particular, ΔFosB in the nucleus accumbens is critical for the reinforcing effects of sexual reward. Research on the interaction between natural and drug rewards suggests that dopaminergic psychostimulants (e.g., amphetamine) and sexual behavior act on similar biomolecular mechanisms to induce ΔFosB in the nucleus accumbens and possess bidirectional reward cross-sensitization effects that are mediated through ΔFosB. This phenomenon is notable since, in humans, a dopamine dysregulation syndrome, characterized by drug-induced compulsive engagement in natural rewards (specifically, sexual activity, shopping, and gambling), has also been observed in some individuals taking dopaminergic medications.
ΔFosB inhibitors (drugs or treatments that oppose its action or reduce its expression) may be an effective treatment for addiction and addictive disorders. Current medical reviews of research involving lab animals have identified a drug class – class I histone deacetylase inhibitors – that indirectly inhibits the function and further increases in the expression of accumbal ΔFosB by inducing G9a expression in the nucleus accumbens after prolonged use. These reviews and subsequent preliminary evidence which used oral administration or intraperitoneal administration of the sodium salt of butyric acid or other class I HDAC inhibitors for an extended period indicate that these drugs have efficacy in reducing addictive behavior in lab animals that have developed addictions to ethanol, psychostimulants (i.e., amphetamine and cocaine), nicotine, and opiates; however, as of August 2015, few clinical trials involving humans with addiction and any HDAC class I inhibitors have been conducted to test for treatment efficacy in humans or identify an optimal dosing regimen.
Plasticity in cocaine addiction
See also: Epigenetics of cocaine addiction
ΔFosB accumulation from excessive drug use
![]() Bottom: this illustrates the progressive increase in ΔFosB expression in the nucleus accumbens following repeated twice daily drug binges, where these phosphorylated (35–37 kilodalton) ΔFosB isoforms persist in the D1-type medium spiny neurons of the nucleus accumbens for up to 2 months. |
ΔFosB levels have been found to increase upon the use of cocaine. Each subsequent dose of cocaine continues to increase ΔFosB levels with no apparent ceiling of tolerance. Elevated levels of ΔFosB leads to increases in brain-derived neurotrophic factor (BDNF) levels, which in turn increases the number of dendritic branches and spines present on neurons involved with the nucleus accumbens and prefrontal cortex areas of the brain. This change can be identified rather quickly, and may be sustained weeks after the last dose of the drug.
Transgenic mice exhibiting inducible expression of ΔFosB primarily in the nucleus accumbens and dorsal striatum exhibit sensitized behavioural responses to cocaine. They self-administer cocaine at lower doses than control, but have a greater likelihood of relapse when the drug is withheld. ΔFosB increases the expression of AMPA receptor subunit GluR2 and also decreases expression of dynorphin, thereby enhancing sensitivity to reward.
Target gene |
Target expression |
Neural effects | Behavioral effects |
---|---|---|---|
c-Fos | ↓ | Molecular switch enabling the chronic induction of ΔFosB |
– |
dynorphin | ↓ |
• Downregulation of κ-opioid feedback loop | • Diminished self-extinguishing response to drug |
NF-κB | ↑ | • Expansion of Nacc dendritic processes • NF-κB inflammatory response in the NAcc • NF-κB inflammatory response in the CPTooltip caudate putamen |
• Increased drug reward • Locomotor sensitization |
GluR2 | ↑ | • Decreased sensitivity to glutamate | • Increased drug reward |
Cdk5 | ↑ | • GluR1 synaptic protein phosphorylation • Expansion of NAcc dendritic processes |
• Decreased drug reward (net effect) |
Summary of addiction-related plasticity
Form of neuroplasticity or behavioral plasticity |
Type of reinforcer | Sources | |||||
---|---|---|---|---|---|---|---|
Opiates | Psychostimulants | High fat or sugar food | Sexual intercourse | Physical exercise (aerobic) |
Environmental enrichment | ||
ΔFosB expression in nucleus accumbens D1-type MSNsTooltip medium spiny neurons |
↑ | ↑ | ↑ | ↑ | ↑ | ↑ | |
Behavioral plasticity | |||||||
Escalation of intake | Yes | Yes | Yes | ||||
Psychostimulant cross-sensitization |
Yes | Not applicable | Yes | Yes | Attenuated | Attenuated | |
Psychostimulant self-administration |
↑ | ↑ | ↓ | ↓ | ↓ | ||
Psychostimulant conditioned place preference |
↑ | ↑ | ↓ | ↑ | ↓ | ↑ | |
Reinstatement of drug-seeking behavior | ↑ | ↑ | ↓ | ↓ | |||
Neurochemical plasticity | |||||||
CREBTooltip cAMP response element-binding protein phosphorylation in the nucleus accumbens |
↓ | ↓ | ↓ | ↓ | ↓ | ||
Sensitized dopamine response in the nucleus accumbens |
No | Yes | No | Yes | |||
Altered striatal dopamine signaling | ↓DRD2, ↑DRD3 | ↑DRD1, ↓DRD2, ↑DRD3 | ↑DRD1, ↓DRD2, ↑DRD3 | ↑DRD2 | ↑DRD2 | ||
Altered striatal opioid signaling | No change or ↑μ-opioid receptors |
↑μ-opioid receptors ↑κ-opioid receptors |
↑μ-opioid receptors | ↑μ-opioid receptors | No change | No change | |
Changes in striatal opioid peptides | ↑dynorphin No change: enkephalin |
↑dynorphin | ↓enkephalin | ↑dynorphin | ↑dynorphin | ||
Mesocorticolimbic synaptic plasticity | |||||||
Number of dendrites in the nucleus accumbens | ↓ | ↑ | ↑ | ||||
Dendritic spine density in the nucleus accumbens |
↓ | ↑ | ↑ |
Other functions in the brain
Viral overexpression of ΔFosB in the output neurons of the nigrostriatal dopamine pathway (i.e., the medium spiny neurons in the dorsal striatum) induces levodopa-induced dyskinesias in animal models of Parkinson's disease. Dorsal striatal ΔFosB is overexpressed in rodents and primates with dyskinesias; postmortem studies of individuals with Parkinson's disease that were treated with levodopa have also observed similar dorsal striatal ΔFosB overexpression. Levetiracetam, an antiepileptic drug, has been shown to dose-dependently decrease the induction of dorsal striatal ΔFosB expression in rats when co-administered with levodopa; the signal transduction involved in this effect is unknown.
ΔFosB expression in the nucleus accumbens shell increases resilience to stress and is induced in this region by acute exposure to social defeat stress.
Antipsychotic drugs have been shown to increase ΔFosB as well, more specifically in the prefrontal cortex. This increase has been found to be part of pathways for the negative side effects that such drugs produce.
See also
Notes
- Synonyms and alternate spellings include: metamfetamine (International Nonproprietary Name (INN)), N-methylamphetamine, desoxyephedrine, Syndrox, and Desoxyn. Common slang terms for methamphetamine include: speed, meth, crystal, crystal meth, glass, shards, ice, and tic and, in New Zealand, "P".
- Enantiomers are molecules that are mirror images of one another; they are structurally identical, but of the opposite orientation.
- ^ The active ingredient in Vicks VapoInhaler is listed as levmetamfetamine, the INN and USAN of levomethamphetamine.
- They suggest consulting with a Certified Poison Control Center on treatment for up-to-date information, advice, and guidance.
- Transcription factors are proteins that increase or decrease the expression of specific genes.
- In simpler terms, this necessary and sufficient relationship means that ΔFosB overexpression in the nucleus accumbens and addiction-related behavioral and neural adaptations always occur together and never occur alone.
- The associated research only involved amphetamine, not methamphetamine; however, this statement is included here due to the similarity between the pharmacodynamics and aphrodisiac effects of amphetamine and methamphetamine.
- NMDA receptors are voltage-dependent ligand-gated ion channels that requires simultaneous binding of glutamate and a co-agonist (D-serine or glycine) to open the ion channel.
- In simplest terms, this means that when either amphetamine or sex is perceived as "more alluring or desirable" through reward sensitization, this effect occurs with the other as well.
- Inhibitors of class I histone deacetylase (HDAC) enzymes are drugs that inhibit four specific histone-modifying enzymes: HDAC1, HDAC2, HDAC3, and HDAC8. Most of the animal research with HDAC inhibitors has been conducted with four drugs: butyrate salts (mainly sodium butyrate), trichostatin A, valproic acid, and SAHA; butyric acid is a naturally occurring short-chain fatty acid in humans, while the latter two compounds are FDA-approved drugs with medical indications unrelated to addiction.
- Specifically, prolonged administration of a class I HDAC inhibitor appears to reduce an animal's motivation to acquire and use an addictive drug without affecting an animals motivation to attain other rewards (i.e., it does not appear to cause motivational anhedonia) and reduce the amount of the drug that is self-administered when it is readily available.
- Among the few clinical trials that employed a class I HDAC inhibitor, one utilized valproate for methamphetamine addiction.
- In other words, c-Fos repression allows ΔFosB to accumulate within nucleus accumbens medium spiny neurons more rapidly because it is selectively induced in this state.
- ΔFosB has been implicated in causing both increases and decreases in dynorphin expression in different studies; this table entry reflects only a decrease.
- Image legend
- Ion channel G proteins & linked receptors (Text color) Transcription factors
- Ion channel G proteins & linked receptors (Text color) Transcription factors
References
- ^ "Toxicity". Methamphetamine. National Center for Biotechnology Information. Retrieved 31 December 2013.
{{cite encyclopedia}}
:|work=
ignored (help) - ^ Cite error: The named reference
Schep
was invoked but never defined (see the help page). - "Adderall XR Prescribing Information" (PDF). United States Food and Drug Administration. December 2013. pp. 12–13. Retrieved 30 December 2013.
- Lemke TL, Williams DA, Roche VF, Zito W (2013). Foye's Principles of Medicinal Chemistry (7th ed.). Philadelphia: Wolters Kluwer Health/Lippincott Williams & Wilkins. p. 648. ISBN 1609133455.
Alternatively, direct oxidation of amphetamine by DA β-hydroxylase can afford norephedrine.
{{cite book}}
: CS1 maint: multiple names: authors list (link) - Krueger SK, Williams DE (June 2005). "Mammalian flavin-containing monooxygenases: structure/function, genetic polymorphisms and role in drug metabolism". Pharmacol. Ther. 106 (3): 357–387. doi:10.1016/j.pharmthera.2005.01.001. PMC 1828602. PMID 15922018.
- Cite error: The named reference
Benzoic1
was invoked but never defined (see the help page). - Cite error: The named reference
Benzoic2
was invoked but never defined (see the help page). - "Properties: Predicted – EP|Suite". Methmphetamine. Retrieved 3 January 2013.
{{cite encyclopedia}}
:|work=
ignored (help) - "Chemical and Physical Properties". Methamphetamine. National Center for Biotechnology Information. Retrieved 31 December 2013.
{{cite encyclopedia}}
:|work=
ignored (help) - "Methamphetamine". Drug profiles. European Monitoring Centre for Drugs and Drug Addiction (EMCDDA). 16 August 2010. Retrieved 1 September 2011.
- "Identification". Methamphetamine. University of Alberta. 8 February 2013. Retrieved 31 December 2013.
{{cite encyclopedia}}
:|work=
ignored (help) - "Meth Slang Names". MethhelpOnline. Retrieved 1 January 2014.
- http://www.police.govt.nz/advice/drugs-and-alcohol/methamphetamine-and-law
- ^ San Francisco Meth Zombies (TV documentary). National Geographic Channel. August 2013. ASIN B00EHAOBAO.
- ^ "Desoxyn Prescribing Information" (PDF). United States Food and Drug Administration. December 2013. Retrieved 6 January 2014.
- Hart, Carl; Marvin, Caroline; Silver, Rae; Smith, Edward (16 November 2011). "Is Cognitive Functioning Impaired in Methamphetamine Users? A Critical Review". Neuropsychopharmacology. 37: 586–608. doi:10.1038/npp.2011.276. PMC 3260986. PMID 22089317. Retrieved 6 March 2015.
- Mitler MM, Hajdukovic R, Erman MK (1993). "Treatment of narcolepsy with methamphetamine". Sleep. 16 (4): 306–317. PMC 2267865. PMID 8341891.
- Morgenthaler TI, Kapur VK, Brown T, Swick TJ, Alessi C, Aurora RN, Boehlecke B, Chesson AL Jr, Friedman L, Maganti R, Owens J, Pancer J, Zak R, Standards of Practice Committee of the American Academy of SM (2007). "Practice parameters for the treatment of narcolepsy and other hypersomnias of central origin". Sleep. PMC 2276123. PMID 18246980.
{{cite journal}}
: Vancouver style error: initials in name 14 (help) - "Package Information". Vicks Vapoinhaler. Vicks. Retrieved 2 January 2014.
- "Identification". Levomethamphetamine. National Center for Biotechnology Information. Retrieved 2 January 2014.
{{cite encyclopedia}}
:|work=
ignored (help) - ^ Westfall DP, Westfall TC (2010). "Miscellaneous Sympathomimetic Agonists". In Brunton LL, Chabner BA, Knollmann BC (ed.). Goodman & Gilman's Pharmacological Basis of Therapeutics (12th ed.). New York: McGraw-Hill. ISBN 978-0-07-162442-8.
{{cite book}}
: External link in
(help); Unknown parameter|sectionurl=
|sectionurl=
ignored (|section-url=
suggested) (help)CS1 maint: multiple names: editors list (link) - ^ Chomchai C, Na Manorom N, Watanarungsan P, Yossuck P, Chomchai S (December 2010). "Methamphetamine abuse during pregnancy and its health impact on neonates born at Siriraj Hospital, Bangkok, Thailand. | PubMed". Southeast Asian J. Trop. Med. Public Health. 35 (1): 228–231. PMID 15272773.
{{cite journal}}
: CS1 maint: multiple names: authors list (link) - ^ Winslow BT, Voorhees KI, Pehl KA (2007). "Methamphetamine abuse". American Family Physician. 76 (8): 1169–1174. PMID 17990840.
{{cite journal}}
: CS1 maint: multiple names: authors list (link) - ^ Hussain F, Frare RW, Py Berrios KL (2012). "Drug abuse identification and pain management in dental patients: a case study and literature review". Gen. Dent. 60 (4): 334–345. PMID 22782046.
{{cite journal}}
: CS1 maint: multiple names: authors list (link) - "Methamphetamine Use (Meth Mouth)". American Dental Association. Archived from the original on June 2008. Retrieved December 2006.
{{cite web}}
: Check date values in:|accessdate=
and|archivedate=
(help) - Hart CL, Marvin CB, Silver R, Smith EE (February 2012). "Is cognitive functioning impaired in methamphetamine users? A critical review". Neuropsychopharmacology. 37 (3): 586–608. doi:10.1038/npp.2011.276. PMC 3260986. PMID 22089317.
{{cite journal}}
: CS1 maint: multiple names: authors list (link) - ^ Halkitis PN, Pandey Mukherjee P, Palamar JJ (2008). "Longitudinal Modeling of Methamphetamine Use and Sexual Risk Behaviors in Gay and Bisexual Men". AIDS and Behavior. 13 (4): 783–791. doi:10.1007/s10461-008-9432-y. PMID 18661225.
{{cite journal}}
: CS1 maint: multiple names: authors list (link) - ^ Patrick Moore (June 2005). "We Are Not OK". VillageVoice. Retrieved January 2011.
{{cite web}}
: Check date values in:|accessdate=
(help) - ^ "Methamphetamine Use and Health | UNSW: The University of New South Wales – Faculty of Medicine" (PDF). Archived from the original (PDF) on August 2008. Retrieved January 2011.
{{cite web}}
: Check date values in:|accessdate=
and|archivedate=
(help) - ^ O'Connor PG (February 2012). "Amphetamines". Merck Manual for Health Care Professionals. Merck. Retrieved 8 May 2012.
- Darke S, Kaye S, McKetin R, Duflou J (May 2008). "Major physical and psychological harms of methamphetamine use". Drug Alcohol Rev. 27 (3): 253–262. doi:10.1080/09595230801923702. PMID 18368606.
{{cite journal}}
: CS1 maint: multiple names: authors list (link) - ^ Beardsley PM, Hauser KF (2014). "Glial modulators as potential treatments of psychostimulant abuse". Adv. Pharmacol. 69: 1–69. doi:10.1016/B978-0-12-420118-7.00001-9. PMC 4103010. PMID 24484974.
Glia (including astrocytes, microglia, and oligodendrocytes), which constitute the majority of cells in the brain, have many of the same receptors as neurons, secrete neurotransmitters and neurotrophic and neuroinflammatory factors, control clearance of neurotransmitters from synaptic clefts, and are intimately involved in synaptic plasticity. Despite their prevalence and spectrum of functions, appreciation of their potential general importance has been elusive since their identification in the mid-1800s, and only relatively recently have they been gaining their due respect. This development of appreciation has been nurtured by the growing awareness that drugs of abuse, including the psychostimulants, affect glial activity, and glial activity, in turn, has been found to modulate the effects of the psychostimulants
- Loftis JM, Janowsky A (2014). "Neuroimmune basis of methamphetamine toxicity". Int. Rev. Neurobiol. 118: 165–197. doi:10.1016/B978-0-12-801284-0.00007-5. PMC 4418472. PMID 25175865.
Collectively, these pathological processes contribute to neurotoxicity (e.g., increased BBB permeability, inflammation, neuronal degeneration, cell death) and neuropsychiatric impairments (e.g., cognitive deficits, mood disorders)
"Figure 7.1: Neuroimmune mechanisms of methamphetamine-induced CNS toxicity" - Cite error: The named reference
Sigma
was invoked but never defined (see the help page). - ^ Malenka RC, Nestler EJ, Hyman SE (2009). "15". In Sydor A, Brown RY (ed.). Molecular Neuropharmacology: A Foundation for Clinical Neuroscience (2nd ed.). New York: McGraw-Hill Medical. p. 370. ISBN 978-0-07-148127-4.
Unlike cocaine and amphetamine, methamphetamine is directly toxic to midbrain dopamine neurons.
{{cite book}}
: CS1 maint: multiple names: authors list (link) - ^ Cruickshank CC, Dyer KR (July 2009). "A review of the clinical pharmacology of methamphetamine". Addiction. 104 (7): 1085–1099. doi:10.1111/j.1360-0443.2009.02564.x. PMID 19426289.
- Thrash B, Thiruchelvan K, Ahuja M, Suppiramaniam V, Dhanasekaran M (2009). "Methamphetamine-induced neurotoxicity: the road to Parkinson's disease" (PDF). Pharmacol Rep. 61 (6): 966–977. doi:10.1016/s1734-1140(09)70158-6. PMID 20081231.
{{cite journal}}
: CS1 maint: multiple names: authors list (link) - Sulzer D, Zecca L (February 2000). "Intraneuronal dopamine-quinone synthesis: a review". Neurotox. Res. 1 (3): 181–195. doi:10.1007/BF03033289. PMID 12835101.
- Miyazaki I, Asanuma M (June 2008). "Dopaminergic neuron-specific oxidative stress caused by dopamine itself". Acta Med. Okayama. 62 (3): 141–150. PMID 18596830.
- ^ Krasnova IN, Cadet JL (May 2009). "Methamphetamine toxicity and messengers of death". Brain Res. Rev. 60 (2): 379–407. doi:10.1016/j.brainresrev.2009.03.002. PMC 2731235. PMID 19328213.
Neuroimaging studies have revealed that METH can indeed cause neurodegenerative changes in the brains of human addicts (Aron and Paulus, 2007; Chang et al., 2007). These abnormalities include persistent decreases in the levels of dopamine transporters (DAT) in the orbitofrontal cortex, dorsolateral prefrontal cortex, and the caudate-putamen (McCann et al., 1998, 2008; Sekine et al., 2003; Volkow et al., 2001a, 2001c). The density of serotonin transporters (5-HTT) is also decreased in the midbrain, caudate, putamen, hypothalamus, thalamus, the orbitofrontal, temporal, and cingulate cortices of METH-dependent individuals (Sekine et al., 2006) ...
Neuropsychological studies have detected deficits in attention, working memory, and decision-making in chronic METH addicts ...
There is compelling evidence that the negative neuropsychiatric consequences of METH abuse are due, at least in part, to drug-induced neuropathological changes in the brains of these METH-exposed individuals ...
Structural magnetic resonance imaging (MRI) studies in METH addicts have revealed substantial morphological changes in their brains. These include loss of gray matter in the cingulate, limbic and paralimbic cortices, significant shrinkage of hippocampi, and hypertrophy of white matter (Thompson et al., 2004). In addition, the brains of METH abusers show evidence of hyperintensities in white matter (Bae et al., 2006; Ernst et al., 2000), decreases in the neuronal marker, N-acetylaspartate (Ernst et al., 2000; Sung et al., 2007), reductions in a marker of metabolic integrity, creatine (Sekine et al., 2002) and increases in a marker of glial activation, myoinositol (Chang et al., 2002; Ernst et al., 2000; Sung et al., 2007; Yen et al., 1994). Elevated choline levels, which are indicative of increased cellular membrane synthesis and turnover are also evident in the frontal gray matter of METH abusers (Ernst et al., 2000; Salo et al., 2007; Taylor et al., 2007). - Yuan J, Hatzidimitriou G, Suthar P, Mueller M, McCann U, Ricaurte G (March 2006). "Relationship between temperature, dopaminergic neurotoxicity, and plasma drug concentrations in methamphetamine-treated squirrel monkeys". The Journal of Pharmacology and Experimental Therapeutics. 316 (3): 1210–1218. doi:10.1124/jpet.105.096503. PMID 16293712.
{{cite journal}}
: CS1 maint: multiple names: authors list (link) - Albertson TE (2011). "Amphetamines". In Olson KR, Anderson IB, Benowitz NL, Blanc PD, Kearney TE, Kim-Katz SY, Wu AHB (ed.). Poisoning & Drug Overdose (6th ed.). New York: McGraw-Hill Medical. pp. 77–79. ISBN 978-0-07-166833-0.
{{cite book}}
: CS1 maint: multiple names: editors list (link) - Oskie SM, Rhee JW (11 February 2011). "Amphetamine Poisoning". Emergency Central. Unbound Medicine. Retrieved 11 June 2013.
- Isbister GK, Buckley NA, Whyte IM (September 2007). "Serotonin toxicity: a practical approach to diagnosis and treatment" (PDF). Med. J. Aust. 187 (6): 361–365. PMID 17874986.
{{cite journal}}
: CS1 maint: multiple names: authors list (link) - ^ Shoptaw SJ, Kao U, Ling W (2009). Shoptaw SJ, Ali R (ed.). "Treatment for amphetamine psychosis". Cochrane Database Syst. Rev. (1): CD003026. doi:10.1002/14651858.CD003026.pub3. PMID 19160215.
A minority of individuals who use amphetamines develop full-blown psychosis requiring care at emergency departments or psychiatric hospitals. In such cases, symptoms of amphetamine psychosis commonly include paranoid and persecutory delusions as well as auditory and visual hallucinations in the presence of extreme agitation. More common (about 18%) is for frequent amphetamine users to report psychotic symptoms that are sub-clinical and that do not require high-intensity intervention ...
About 5–15% of the users who develop an amphetamine psychosis fail to recover completely (Hofmann 1983) ...
Findings from one trial indicate use of antipsychotic medications effectively resolves symptoms of acute amphetamine psychosis.{{cite journal}}
: CS1 maint: multiple names: authors list (link) - Hofmann FG (1983). A Handbook on Drug and Alcohol Abuse: The Biomedical Aspects (2nd ed.). New York: Oxford University Press. p. 329. ISBN 978-0-19-503057-0.
- Berman SM, Kuczenski R, McCracken JT, London ED (February 2009). "Potential adverse effects of amphetamine treatment on brain and behavior: a review". Mol. Psychiatry. 14 (2): 123–142. doi:10.1038/mp.2008.90. PMC 2670101. PMID 18698321.
{{cite journal}}
: CS1 maint: multiple names: authors list (link) - ^ Malenka RC, Nestler EJ, Hyman SE (2009). "Chapter 15: Reinforcement and Addictive Disorders". In Sydor A, Brown RY (eds.). Molecular Neuropharmacology: A Foundation for Clinical Neuroscience (2nd ed.). New York: McGraw-Hill Medical. pp. 364–375. ISBN 9780071481274. Cite error: The named reference "Addiction glossary" was defined multiple times with different content (see the help page).
- ^ Nestler EJ (December 2013). "Cellular basis of memory for addiction". Dialogues in Clinical Neuroscience. 15 (4): 431–443. PMC 3898681. PMID 24459410.
Despite the importance of numerous psychosocial factors, at its core, drug addiction involves a biological process: the ability of repeated exposure to a drug of abuse to induce changes in a vulnerable brain that drive the compulsive seeking and taking of drugs, and loss of control over drug use, that define a state of addiction. ... A large body of literature has demonstrated that such ΔFosB induction in D1-type neurons increases an animal's sensitivity to drug as well as natural rewards and promotes drug self-administration, presumably through a process of positive reinforcement ... Another ΔFosB target is cFos: as ΔFosB accumulates with repeated drug exposure it represses c-Fos and contributes to the molecular switch whereby ΔFosB is selectively induced in the chronic drug-treated state.. ... Moreover, there is increasing evidence that, despite a range of genetic risks for addiction across the population, exposure to sufficiently high doses of a drug for long periods of time can transform someone who has relatively lower genetic loading into an addict.
Cite error: The named reference "Cellular basis" was defined multiple times with different content (see the help page). - ^ Volkow ND, Koob GF, McLellan AT (January 2016). "Neurobiologic Advances from the Brain Disease Model of Addiction". New England Journal of Medicine. 374 (4): 363–371. doi:10.1056/NEJMra1511480. PMC 6135257. PMID 26816013.
Substance-use disorder: A diagnostic term in the fifth edition of the Diagnostic and Statistical Manual of Mental Disorders (DSM-5) referring to recurrent use of alcohol or other drugs that causes clinically and functionally significant impairment, such as health problems, disability, and failure to meet major responsibilities at work, school, or home. Depending on the level of severity, this disorder is classified as mild, moderate, or severe.
Addiction: A term used to indicate the most severe, chronic stage of substance-use disorder, in which there is a substantial loss of self-control, as indicated by compulsive drug taking despite the desire to stop taking the drug. In the DSM-5, the term addiction is synonymous with the classification of severe substance-use disorder. - ^ Renthal W, Nestler EJ (September 2009). "Chromatin regulation in drug addiction and depression". Dialogues in Clinical Neuroscience. 11 (3): 257–268. doi:10.31887/DCNS.2009.11.3/wrenthal. PMC 2834246. PMID 19877494.
increase cAMP levels in striatum, which activates protein kinase A (PKA) and leads to phosphorylation of its targets. This includes the cAMP response element binding protein (CREB), the phosphorylation of which induces its association with the histone acetyltransferase, CREB binding protein (CBP) to acetylate histones and facilitate gene activation. This is known to occur on many genes including fosB and c-fos in response to psychostimulant exposure. ΔFosB is also upregulated by chronic psychostimulant treatments, and is known to activate certain genes (eg, cdk5) and repress others (eg, c-fos) where it recruits HDAC1 as a corepressor. ... Chronic exposure to psychostimulants increases glutamatergic from the prefrontal cortex to the NAc. Glutamatergic signaling elevates Ca2+ levels in NAc postsynaptic elements where it activates CaMK (calcium/calmodulin protein kinases) signaling, which, in addition to phosphorylating CREB, also phosphorylates HDAC5.
Figure 2: Psychostimulant-induced signaling events - ^ Broussard JI (January 2012). "Co-transmission of dopamine and glutamate". The Journal of General Physiology. 139 (1): 93–96. doi:10.1085/jgp.201110659. PMC 3250102. PMID 22200950.
Coincident and convergent input often induces plasticity on a postsynaptic neuron. The NAc integrates processed information about the environment from basolateral amygdala, hippocampus, and prefrontal cortex (PFC), as well as projections from midbrain dopamine neurons. Previous studies have demonstrated how dopamine modulates this integrative process. For example, high frequency stimulation potentiates hippocampal inputs to the NAc while simultaneously depressing PFC synapses (Goto and Grace, 2005). The converse was also shown to be true; stimulation at PFC potentiates PFC–NAc synapses but depresses hippocampal–NAc synapses. In light of the new functional evidence of midbrain dopamine/glutamate co-transmission (references above), new experiments of NAc function will have to test whether midbrain glutamatergic inputs bias or filter either limbic or cortical inputs to guide goal-directed behavior.
- ^ Kanehisa Laboratories (10 October 2014). "Amphetamine – Homo sapiens (human)". KEGG Pathway. Retrieved 31 October 2014.
Most addictive drugs increase extracellular concentrations of dopamine (DA) in nucleus accumbens (NAc) and medial prefrontal cortex (mPFC), projection areas of mesocorticolimbic DA neurons and key components of the "brain reward circuit". Amphetamine achieves this elevation in extracellular levels of DA by promoting efflux from synaptic terminals. ... Chronic exposure to amphetamine induces a unique transcription factor delta FosB, which plays an essential role in long-term adaptive changes in the brain.
- ^ Cadet JL, Brannock C, Jayanthi S, Krasnova IN (2015). "Transcriptional and epigenetic substrates of methamphetamine addiction and withdrawal: evidence from a long-access self-administration model in the rat". Molecular Neurobiology. 51 (2): 696–717 (Figure 1). doi:10.1007/s12035-014-8776-8. PMC 4359351. PMID 24939695.
- ^ Robison AJ, Nestler EJ (November 2011). "Transcriptional and epigenetic mechanisms of addiction". Nature Reviews Neuroscience. 12 (11): 623–637. doi:10.1038/nrn3111. PMC 3272277. PMID 21989194.
ΔFosB serves as one of the master control proteins governing this structural plasticity. ... ΔFosB also represses G9a expression, leading to reduced repressive histone methylation at the cdk5 gene. The net result is gene activation and increased CDK5 expression. ... In contrast, ΔFosB binds to the c-fos gene and recruits several co-repressors, including HDAC1 (histone deacetylase 1) and SIRT 1 (sirtuin 1). ... The net result is c-fos gene repression.
Figure 4: Epigenetic basis of drug regulation of gene expression - ^ Nestler EJ (December 2012). "Transcriptional mechanisms of drug addiction". Clinical Psychopharmacology and Neuroscience. 10 (3): 136–143. doi:10.9758/cpn.2012.10.3.136. PMC 3569166. PMID 23430970.
The 35-37 kD ΔFosB isoforms accumulate with chronic drug exposure due to their extraordinarily long half-lives. ... As a result of its stability, the ΔFosB protein persists in neurons for at least several weeks after cessation of drug exposure. ... ΔFosB overexpression in nucleus accumbens induces NFκB ... In contrast, the ability of ΔFosB to repress the c-Fos gene occurs in concert with the recruitment of a histone deacetylase and presumably several other repressive proteins such as a repressive histone methyltransferase
- ^ Nestler EJ (October 2008). "Transcriptional mechanisms of addiction: Role of ΔFosB". Philosophical Transactions of the Royal Society B: Biological Sciences. 363 (1507): 3245–3255. doi:10.1098/rstb.2008.0067. PMC 2607320. PMID 18640924.
Recent evidence has shown that ΔFosB also represses the c-fos gene that helps create the molecular switch—from the induction of several short-lived Fos family proteins after acute drug exposure to the predominant accumulation of ΔFosB after chronic drug exposure
- ^ Hyman SE, Malenka RC, Nestler EJ (July 2006). "Neural mechanisms of addiction: the role of reward-related learning and memory". Annu. Rev. Neurosci. 29: 565–598. doi:10.1146/annurev.neuro.29.051605.113009. PMID 16776597.
{{cite journal}}
: CS1 maint: multiple names: authors list (link) Cite error: The named reference "Nestler, Hyman, and Malenka 2" was defined multiple times with different content (see the help page). - ^ Robison AJ, Nestler EJ (November 2011). "Transcriptional and epigenetic mechanisms of addiction". Nat. Rev. Neurosci. 12 (11): 623–637. doi:10.1038/nrn3111. PMC 3272277. PMID 21989194.
ΔFosB has been linked directly to several addiction-related behaviors ... Importantly, genetic or viral overexpression of ΔJunD, a dominant negative mutant of JunD which antagonizes ΔFosB- and other AP-1-mediated transcriptional activity, in the NAc or OFC blocks these key effects of drug exposure. This indicates that ΔFosB is both necessary and sufficient for many of the changes wrought in the brain by chronic drug exposure. ΔFosB is also induced in D1-type NAc MSNs by chronic consumption of several natural rewards, including sucrose, high fat food, sex, wheel running, where it promotes that consumption. This implicates ΔFosB in the regulation of natural rewards under normal conditions and perhaps during pathological addictive-like states.
Cite error: The named reference "Nestler" was defined multiple times with different content (see the help page). - Malenka RC, Nestler EJ, Hyman SE (2009). "Chapter 4: Signal Transduction in the Brain". In Sydor A, Brown RY (ed.). Molecular Neuropharmacology: A Foundation for Clinical Neuroscience (2nd ed.). New York, USA: McGraw-Hill Medical. p. 94. ISBN 9780071481274.
{{cite book}}
: CS1 maint: multiple names: authors list (link) - ^ Ruffle JK (November 2014). "Molecular neurobiology of addiction: what's all the (Δ)FosB about?". Am. J. Drug Alcohol Abuse. 40 (6): 428–437. doi:10.3109/00952990.2014.933840. PMID 25083822.
ΔFosB is an essential transcription factor implicated in the molecular and behavioral pathways of addiction following repeated drug exposure.
Cite error: The named reference "What the ΔFosB?" was defined multiple times with different content (see the help page). - ^ Olsen CM (December 2011). "Natural rewards, neuroplasticity, and non-drug addictions". Neuropharmacology. 61 (7): 1109–1122. doi:10.1016/j.neuropharm.2011.03.010. PMC 3139704. PMID 21459101.
Similar to environmental enrichment, studies have found that exercise reduces self-administration and relapse to drugs of abuse (Cosgrove et al., 2002; Zlebnik et al., 2010). There is also some evidence that these preclinical findings translate to human populations, as exercise reduces withdrawal symptoms and relapse in abstinent smokers (Daniel et al., 2006; Prochaska et al., 2008), and one drug recovery program has seen success in participants that train for and compete in a marathon as part of the program (Butler, 2005). ... In humans, the role of dopamine signaling in incentive-sensitization processes has recently been highlighted by the observation of a dopamine dysregulation syndrome in some patients taking dopaminergic drugs. This syndrome is characterized by a medication-induced increase in (or compulsive) engagement in non-drug rewards such as gambling, shopping, or sex (Evans et al., 2006; Aiken, 2007; Lader, 2008).
Cite error: The named reference "Natural and drug addictions" was defined multiple times with different content (see the help page). - ^ Kanehisa Laboratories (29 October 2014). "Alcoholism – Homo sapiens (human)". KEGG Pathway. Retrieved 31 October 2014. Cite error: The named reference "Alcoholism ΔFosB" was defined multiple times with different content (see the help page).
- ^ Kim Y, Teylan MA, Baron M, Sands A, Nairn AC, Greengard P (February 2009). "Methylphenidate-induced dendritic spine formation and DeltaFosB expression in nucleus accumbens". Proc. Natl. Acad. Sci. U.S.A. 106 (8): 2915–2920. doi:10.1073/pnas.0813179106. PMC 2650365. PMID 19202072. Cite error: The named reference "MPH ΔFosB" was defined multiple times with different content (see the help page).
- ^ Nestler EJ (January 2014). "Epigenetic mechanisms of drug addiction". Neuropharmacology. 76 Pt B: 259–268. doi:10.1016/j.neuropharm.2013.04.004. PMC 3766384. PMID 23643695. Cite error: The named reference "Nestler 2014 epigenetics" was defined multiple times with different content (see the help page).
- ^ Blum K, Werner T, Carnes S, Carnes P, Bowirrat A, Giordano J, Oscar-Berman M, Gold M (March 2012). "Sex, drugs, and rock 'n' roll: hypothesizing common mesolimbic activation as a function of reward gene polymorphisms". J. Psychoactive Drugs. 44 (1): 38–55. doi:10.1080/02791072.2012.662112. PMC 4040958. PMID 22641964.
It has been found that deltaFosB gene in the NAc is critical for reinforcing effects of sexual reward. Pitchers and colleagues (2010) reported that sexual experience was shown to cause DeltaFosB accumulation in several limbic brain regions including the NAc, medial pre-frontal cortex, VTA, caudate, and putamen, but not the medial preoptic nucleus. ... these findings support a critical role for DeltaFosB expression in the NAc in the reinforcing effects of sexual behavior and sexual experience-induced facilitation of sexual performance. ... both drug addiction and sexual addiction represent pathological forms of neuroplasticity along with the emergence of aberrant behaviors involving a cascade of neurochemical changes mainly in the brain's rewarding circuitry.
{{cite journal}}
: CS1 maint: multiple names: authors list (link) Cite error: The named reference "ΔFosB reward" was defined multiple times with different content (see the help page). - ^ Pitchers KK, Vialou V, Nestler EJ, Laviolette SR, Lehman MN, Coolen LM (February 2013). "Natural and drug rewards act on common neural plasticity mechanisms with ΔFosB as a key mediator". J. Neurosci. 33 (8): 3434–3442. doi:10.1523/JNEUROSCI.4881-12.2013. PMC 3865508. PMID 23426671.
Drugs of abuse induce neuroplasticity in the natural reward pathway, specifically the nucleus accumbens (NAc), thereby causing development and expression of addictive behavior. ... Together, these findings demonstrate that drugs of abuse and natural reward behaviors act on common molecular and cellular mechanisms of plasticity that control vulnerability to drug addiction, and that this increased vulnerability is mediated by ΔFosB and its downstream transcriptional targets. ... Sexual behavior is highly rewarding (Tenk et al., 2009), and sexual experience causes sensitized drug-related behaviors, including cross-sensitization to amphetamine (Amph)-induced locomotor activity (Bradley and Meisel, 2001; Pitchers et al., 2010a) and enhanced Amph reward (Pitchers et al., 2010a). Moreover, sexual experience induces neural plasticity in the NAc similar to that induced by psychostimulant exposure, including increased dendritic spine density (Meisel and Mullins, 2006; Pitchers et al., 2010a), altered glutamate receptor trafficking, and decreased synaptic strength in prefrontal cortex-responding NAc shell neurons (Pitchers et al., 2012). Finally, periods of abstinence from sexual experience were found to be critical for enhanced Amph reward, NAc spinogenesis (Pitchers et al., 2010a), and glutamate receptor trafficking (Pitchers et al., 2012). These findings suggest that natural and drug reward experiences share common mechanisms of neural plasticity
{{cite journal}}
: CS1 maint: multiple names: authors list (link) Cite error: The named reference "Amph and sex addiction" was defined multiple times with different content (see the help page). - Malenka RC, Nestler EJ, Hyman SE (2009). "Chapter 15: Reinforcement and Addictive Disorders". In Sydor A, Brown RY (ed.). Molecular Neuropharmacology: A Foundation for Clinical Neuroscience (2nd ed.). New York, USA: McGraw-Hill Medical. p. 386. ISBN 9780071481274.
Currently, cognitive–behavioral therapies are the most successful treatment available for preventing the relapse of psychostimulant use.
{{cite book}}
: CS1 maint: multiple names: authors list (link) - Stoops WW, Rush CR (May 2014). "Combination pharmacotherapies for stimulant use disorder: a review of clinical findings and recommendations for future research". Expert Rev Clin Pharmacol. 7 (3): 363–374. doi:10.1586/17512433.2014.909283. PMID 24716825.
Despite concerted efforts to identify a pharmacotherapy for managing stimulant use disorders, no widely effective medications have been approved.
- Perez-Mana C, Castells X, Torrens M, Capella D, Farre M (September 2013). "Efficacy of psychostimulant drugs for amphetamine abuse or dependence". Cochrane Database Syst. Rev. 9: CD009695. doi:10.1002/14651858.CD009695.pub2. PMID 23996457.
To date, no pharmacological treatment has been approved for , and psychotherapy remains the mainstay of treatment. ... Results of this review do not support the use of psychostimulant medications at the tested doses as a replacement therapy
- Forray A, Sofuoglu M (February 2014). "Future pharmacological treatments for substance use disorders". Br. J. Clin. Pharmacol. 77 (2): 382–400. doi:10.1111/j.1365-2125.2012.04474.x. PMC 4014020. PMID 23039267.
- ^ Malenka RC, Nestler EJ, Hyman SE (2009). "Chapter 5: Excitatory and Inhibitory Amino Acids". In Sydor A, Brown RY (ed.). Molecular Neuropharmacology: A Foundation for Clinical Neuroscience (2nd ed.). New York, USA: McGraw-Hill Medical. pp. 124–125. ISBN 9780071481274.
At membrane potentials more negative than approximately −50 mV, the Mg2+ in the extracellular fluid of the brain virtually abolishes ion flux through NMDA receptor channels, even in the presence of glutamate. ... The NMDA receptor is unique among all neurotransmitter receptors in that its activation requires the simultaneous binding of two different agonists. In addition to the binding of glutamate at the conventional agonist-binding site, the binding of glycine appears to be required for receptor activation. Because neither of these agonists alone can open this ion channel, glutamate and glycine are referred to as coagonists of the NMDA receptor. The physiologic significance of the glycine binding site is unclear because the normal extracellular concentration of glycine is believed to be saturating. However, recent evidence suggests that D-serine may be the endogenous agonist for this site.
{{cite book}}
: CS1 maint: multiple names: authors list (link) - Nechifor M (March 2008). "Magnesium in drug dependences". Magnes. Res. 21 (1): 5–15. PMID 18557129.
- O'Connor, Patrick. "Amphetamines: Drug Use and Abuse". Merck Manual Home Health Handbook. Merck. Retrieved 26 September 2013.
- Pérez-Mañá C, Castells X, Torrens M, Capellà D, Farre M (2013). Pérez-Mañá, Clara (ed.). "Efficacy of psychostimulant drugs for amphetamine abuse or dependence". Cochrane Database Syst. Rev. 9: CD009695. doi:10.1002/14651858.CD009695.pub2. PMID 23996457.
{{cite journal}}
: CS1 maint: multiple names: authors list (link) - ^ Shoptaw SJ, Kao U, Heinzerling K, Ling W (2009). Shoptaw SJ (ed.). "Treatment for amphetamine withdrawal". Cochrane Database Syst. Rev. (2): CD003021. doi:10.1002/14651858.CD003021.pub2. PMID 19370579.
The prevalence of this withdrawal syndrome is extremely common (Cantwell 1998; Gossop 1982) with 87.6% of 647 individuals with amphetamine dependence reporting six or more signs of amphetamine withdrawal listed in the DSM when the drug is not available (Schuckit 1999) ... Withdrawal symptoms typically present within 24 hours of the last use of amphetamine, with a withdrawal syndrome involving two general phases that can last 3 weeks or more. The first phase of this syndrome is the initial "crash" that resolves within about a week (Gossop 1982;McGregor 2005)
{{cite journal}}
: CS1 maint: multiple names: authors list (link) - ^ "Entrez Gene: FOSB FBJ murine osteosarcoma viral oncogene homolog B".
- Siderovski DP, Blum S, Forsdyke RE, Forsdyke DR (October 1990). "A set of human putative lymphocyte G0/G1 switch genes includes genes homologous to rodent cytokine and zinc finger protein-encoding genes". DNA and Cell Biology. 9 (8): 579–87. doi:10.1089/dna.1990.9.579. PMID 1702972.
- Martin-Gallardo A, McCombie WR, Gocayne JD, FitzGerald MG, Wallace S, Lee BM, Lamerdin J, Trapp S, Kelley JM, Liu LI (April 1992). "Automated DNA sequencing and analysis of 106 kilobases from human chromosome 19q13.3". Nature Genetics. 1 (1): 34–9. doi:10.1038/ng0492-34. PMID 1301997. S2CID 1986255.
- Sabatakos G, Rowe GC, Kveiborg M, Wu M, Neff L, Chiusaroli R, Philbrick WM, Baron R (May 2008). "Doubly truncated FosB isoform (Delta2DeltaFosB) induces osteosclerosis in transgenic mice and modulates expression and phosphorylation of Smads in osteoblasts independent of intrinsic AP-1 activity". Journal of Bone and Mineral Research. 23 (5): 584–95. doi:10.1359/jbmr.080110. PMC 2674536. PMID 18433296.
- ^ Biliński P, Wojtyła A, Kapka-Skrzypczak L, Chwedorowicz R, Cyranka M, Studziński T (2012). "Epigenetic regulation in drug addiction". Annals of Agricultural and Environmental Medicine. 19 (3): 491–6. PMID 23020045.
For these reasons, ΔFosB is considered a primary and causative transcription factor in creating new neural connections in the reward centre, prefrontal cortex, and other regions of the limbic system. This is reflected in the increased, stable and long-lasting level of sensitivity to cocaine and other drugs, and tendency to relapse even after long periods of abstinence. These newly constructed networks function very efficiently via new pathways as soon as drugs of abuse are further taken ... In this way, the induction of CDK5 gene expression occurs together with suppression of the G9A gene coding for dimethyltransferase acting on the histone H3. A feedback mechanism can be observed in the regulation of these 2 crucial factors that determine the adaptive epigenetic response to cocaine. This depends on ΔFosB inhibiting G9a gene expression, i.e. H3K9me2 synthesis which in turn inhibits transcription factors for ΔFosB. For this reason, the observed hyper-expression of G9a, which ensures high levels of the dimethylated form of histone H3, eliminates the neuronal structural and plasticity effects caused by cocaine by means of this feedback which blocks ΔFosB transcription
- Ohnishi YN, Ohnishi YH, Vialou V, Mouzon E, LaPlant Q, Nishi A, Nestler EJ (January 2015). "Functional role of the N-terminal domain of ΔFosB in response to stress and drugs of abuse". Neuroscience. 284: 165–70. doi:10.1016/j.neuroscience.2014.10.002. PMC 4268105. PMID 25313003.
- Nakabeppu Y, Nathans D (February 1991). "A naturally occurring truncated form of FosB that inhibits Fos/Jun transcriptional activity". Cell. 64 (4): 751–9. doi:10.1016/0092-8674(91)90504-R. PMID 1900040. S2CID 23904956.
- ^ Nestler EJ (October 2008). "Review. Transcriptional mechanisms of addiction: role of DeltaFosB". Philosophical Transactions of the Royal Society of London. Series B, Biological Sciences. 363 (1507): 3245–55. doi:10.1098/rstb.2008.0067. PMC 2607320. PMID 18640924.
Recent evidence has shown that ΔFosB also represses the c-fos gene that helps create the molecular switch—from the induction of several short-lived Fos family proteins after acute drug exposure to the predominant accumulation of ΔFosB after chronic drug exposure—cited earlier (Renthal et al. in press). The mechanism responsible for ΔFosB repression of c-fos expression is complex and is covered below. ...
Examples of validated targets for ΔFosB in nucleus accumbens ... GluR2 ... dynorphin ... Cdk5 ... NFκB ... c-Fos
Table 3 - Renthal W, Nestler EJ (August 2008). "Epigenetic mechanisms in drug addiction". Trends in Molecular Medicine. 14 (8): 341–50. doi:10.1016/j.molmed.2008.06.004. PMC 2753378. PMID 18635399.
- Renthal W, Kumar A, Xiao G, Wilkinson M, Covington HE, Maze I, Sikder D, Robison AJ, LaPlant Q, Dietz DM, Russo SJ, Vialou V, Chakravarty S, Kodadek TJ, Stack A, Kabbaj M, Nestler EJ (May 2009). "Genome-wide analysis of chromatin regulation by cocaine reveals a role for sirtuins". Neuron. 62 (3): 335–48. doi:10.1016/j.neuron.2009.03.026. PMC 2779727. PMID 19447090.
- Sabatakos G, Sims NA, Chen J, Aoki K, Kelz MB, Amling M, Bouali Y, Mukhopadhyay K, Ford K, Nestler EJ, Baron R (September 2000). "Overexpression of DeltaFosB transcription factor(s) increases bone formation and inhibits adipogenesis". Nature Medicine. 6 (9): 985–90. doi:10.1038/79683. PMID 10973317. S2CID 20302360.
- ^ Nestler EJ, Barrot M, Self DW (September 2001). "DeltaFosB: a sustained molecular switch for addiction". Proceedings of the National Academy of Sciences of the United States of America. 98 (20): 11042–6. Bibcode:2001PNAS...9811042N. doi:10.1073/pnas.191352698. PMC 58680. PMID 11572966.
- Salaya-Velazquez NF, López-Muciño LA, Mejía-Chávez S, Sánchez-Aparicio P, Domínguez-Guadarrama AA, Venebra-Muñoz A (February 2020). "Anandamide and sucralose change ΔFosB expression in the reward system". NeuroReport. 31 (3): 240–244. doi:10.1097/WNR.0000000000001400. PMID 31923023. S2CID 210149592.
- Steiner H, Van Waes V (January 2013). "Addiction-related gene regulation: risks of exposure to cognitive enhancers vs. other psychostimulants". Progress in Neurobiology. 100: 60–80. doi:10.1016/j.pneurobio.2012.10.001. PMC 3525776. PMID 23085425.
- Malenka RC, Nestler EJ, Hyman SE (2009). "Chapter 15: Reinforcement and addictive disorders". In Sydor A, Brown RY (eds.). Molecular Neuropharmacology: A Foundation for Clinical Neuroscience (2nd ed.). New York: McGraw-Hill Medical. pp. 384–385. ISBN 9780071481274.
- ^ McCowan TJ, Dhasarathy A, Carvelli L (February 2015). "The Epigenetic Mechanisms of Amphetamine". J. Addict. Prev. 2015 (Suppl 1). PMC 4955852. PMID 27453897.
Epigenetic modifications caused by addictive drugs play an important role in neuronal plasticity and in drug-induced behavioral responses. Although few studies have investigated the effects of AMPH on gene regulation (Table 1), current data suggest that AMPH acts at multiple levels to alter histone/DNA interaction and to recruit transcription factors which ultimately cause repression of some genes and activation of other genes. Importantly, some studies have also correlated the epigenetic regulation induced by AMPH with the behavioral outcomes caused by this drug, suggesting therefore that epigenetics remodeling underlies the behavioral changes induced by AMPH. If this proves to be true, the use of specific drugs that inhibit histone acetylation, methylation or DNA methylation might be an important therapeutic alternative to prevent and/or reverse AMPH addiction and mitigate the side effects generate by AMPH when used to treat ADHD.
- ^ Walker DM, Cates HM, Heller EA, Nestler EJ (February 2015). "Regulation of chromatin states by drugs of abuse". Curr. Opin. Neurobiol. 30: 112–121. doi:10.1016/j.conb.2014.11.002. PMC 4293340. PMID 25486626.
Studies investigating general HDAC inhibition on behavioral outcomes have produced varying results but it seems that the effects are specific to the timing of exposure (either before, during or after exposure to drugs of abuse) as well as the length of exposure
- ^ Primary references involving sodium butyrate:
• Kennedy PJ, Feng J, Robison AJ, Maze I, Badimon A, Mouzon E, Chaudhury D, Damez-Werno DM, Haggarty SJ, Han MH, Bassel-Duby R, Olson EN, Nestler EJ (April 2013). "Class I HDAC inhibition blocks cocaine-induced plasticity by targeted changes in histone methylation". Nat. Neurosci. 16 (4): 434–440. doi:10.1038/nn.3354. PMC 3609040. PMID 23475113.While acute HDAC inhibition enhances the behavioral effects of cocaine or amphetamine, studies suggest that more chronic regimens block psychostimulant-induced plasticity. ... The effects of pharmacological inhibition of HDACs on psychostimulant-induced plasticity appear to depend on the timecourse of HDAC inhibition. Studies employing co-administration procedures in which inhibitors are given acutely, just prior to psychostimulant administration, report heightened behavioral responses to the drug. In contrast, experimental paradigms like the one employed here, in which HDAC inhibitors are administered more chronically, for several days prior to psychostimulant exposure, show inhibited expression or decreased acquisition of behavioral adaptations to drug. The clustering of seemingly discrepant results based on experimental methodologies is interesting in light of our present findings. Both HDAC inhibitors and psychostimulants increase global levels of histone acetylation in NAc. Thus, when co-administered acutely, these drugs may have synergistic effects, leading to heightened transcriptional activation of psychostimulant-regulated target genes. In contrast, when a psychostimulant is given in the context of prolonged, HDAC inhibitor-induced hyperacetylation, homeostatic processes may direct AcH3 binding to the promoters of genes (e.g., G9a) responsible for inducing chromatin condensation and gene repression (e.g., via H3K9me2) in order to dampen already heightened transcriptional activation. Our present findings thus demonstrate clear cross talk among histone PTMs and suggest that decreased behavioral sensitivity to psychostimulants following prolonged HDAC inhibition might be mediated through decreased activity of HDAC1 at H3K9 KMT promoters and subsequent increases in H3K9me2 and gene repression.
• Simon-O'Brien E, Alaux-Cantin S, Warnault V, Buttolo R, Naassila M, Vilpoux C (July 2015). "The histone deacetylase inhibitor sodium butyrate decreases excessive ethanol intake in dependent animals". Addict Biol. 20 (4): 676–689. doi:10.1111/adb.12161. PMID 25041570. S2CID 28667144.Altogether, our results clearly demonstrated the efficacy of NaB in preventing excessive ethanol intake and relapse and support the hypothesis that HDACi may have a potential use in alcohol addiction treatment.
• Castino MR, Cornish JL, Clemens KJ (April 2015). "Inhibition of histone deacetylases facilitates extinction and attenuates reinstatement of nicotine self-administration in rats". PLOS ONE. 10 (4): e0124796. Bibcode:2015PLoSO..1024796C. doi:10.1371/journal.pone.0124796. PMC 4399837. PMID 25880762.treatment with NaB significantly attenuated nicotine and nicotine + cue reinstatement when administered immediately ... These results provide the first demonstration that HDAC inhibition facilitates the extinction of responding for an intravenously self-administered drug of abuse and further highlight the potential of HDAC inhibitors in the treatment of drug addiction.
- Kyzar EJ, Pandey SC (August 2015). "Molecular mechanisms of synaptic remodeling in alcoholism". Neurosci. Lett. 601: 11–9. doi:10.1016/j.neulet.2015.01.051. PMC 4506731. PMID 25623036.
Increased HDAC2 expression decreases the expression of genes important for the maintenance of dendritic spine density such as BDNF, Arc, and NPY, leading to increased anxiety and alcohol-seeking behavior. Decreasing HDAC2 reverses both the molecular and behavioral consequences of alcohol addiction, thus implicating this enzyme as a potential treatment target (Fig. 3). HDAC2 is also crucial for the induction and maintenance of structural synaptic plasticity in other neurological domains such as memory formation . Taken together, these findings underscore the potential usefulness of HDAC inhibition in treating alcohol use disorders ... Given the ability of HDAC inhibitors to potently modulate the synaptic plasticity of learning and memory , these drugs hold potential as treatment for substance abuse-related disorders. ... Our lab and others have published extensively on the ability of HDAC inhibitors to reverse the gene expression deficits caused by multiple models of alcoholism and alcohol abuse, the results of which were discussed above . This data supports further examination of histone modifying agents as potential therapeutic drugs in the treatment of alcohol addiction ... Future studies should continue to elucidate the specific epigenetic mechanisms underlying compulsive alcohol use and alcoholism, as this is likely to provide new molecular targets for clinical intervention.
- Kheirabadi GR, Ghavami M, Maracy MR, Salehi M, Sharbafchi MR (2016). "Effect of add-on valproate on craving in methamphetamine depended patients: A randomized trial". Advanced Biomedical Research. 5: 149. doi:10.4103/2277-9175.187404. PMC 5025910. PMID 27656618.
- Hope BT (May 1998). "Cocaine and the AP-1 transcription factor complex". Annals of the New York Academy of Sciences. 844 (1): 1–6. Bibcode:1998NYASA.844....1H. doi:10.1111/j.1749-6632.1998.tb08216.x. PMID 9668659. S2CID 11683570.
- ^ Kelz MB, Chen J, Carlezon WA, Whisler K, Gilden L, Beckmann AM, Steffen C, Zhang YJ, Marotti L, Self DW, Tkatch T, Baranauskas G, Surmeier DJ, Neve RL, Duman RS, Picciotto MR, Nestler EJ (September 1999). "Expression of the transcription factor deltaFosB in the brain controls sensitivity to cocaine". Nature. 401 (6750): 272–6. Bibcode:1999Natur.401..272K. doi:10.1038/45790. PMID 10499584. S2CID 4390717.
- ^ Colby CR, Whisler K, Steffen C, Nestler EJ, Self DW (March 2003). "Striatal cell type-specific overexpression of DeltaFosB enhances incentive for cocaine". The Journal of Neuroscience. 23 (6): 2488–93. doi:10.1523/JNEUROSCI.23-06-02488.2003. PMC 6742034. PMID 12657709.
- Cao X, Yasuda T, Uthayathas S, Watts RL, Mouradian MM, Mochizuki H, Papa SM (May 2010). "Striatal overexpression of DeltaFosB reproduces chronic levodopa-induced involuntary movements". The Journal of Neuroscience. 30 (21): 7335–43. doi:10.1523/JNEUROSCI.0252-10.2010. PMC 2888489. PMID 20505100.
- ^ Du H, Nie S, Chen G, Ma K, Xu Y, Zhang Z, Papa SM, Cao X (2015). "Levetiracetam Ameliorates L-DOPA-Induced Dyskinesia in Hemiparkinsonian Rats Inducing Critical Molecular Changes in the Striatum". Parkinson's Disease. 2015: 253878. doi:10.1155/2015/253878. PMC 4322303. PMID 25692070.
Furthermore, the transgenic overexpression of ΔFosB reproduces AIMs in hemiparkinsonian rats without chronic exposure to L-DOPA . ... FosB/ΔFosB immunoreactive neurons increased in the dorsolateral part of the striatum on the lesion side with the used antibody that recognizes all members of the FosB family. All doses of levetiracetam decreased the number of FosB/ΔFosB positive cells (from 88.7 ± 1.7/section in the control group to 65.7 ± 0.87, 42.3 ± 1.88, and 25.7 ± 1.2/section in the 15, 30, and 60 mg groups, resp.; Figure 2). These results indicate dose-dependent effects of levetiracetam on FosB/ΔFosB expression. ... In addition, transcription factors expressed with chronic events such as ΔFosB (a truncated splice variant of FosB) are overexpressed in the striatum of rodents and primates with dyskinesias . ... Furthermore, ΔFosB overexpression has been observed in postmortem striatal studies of Parkinsonian patients chronically treated with L-DOPA . ... Of note, the most prominent effect of levetiracetam was the reduction of ΔFosB expression, which cannot be explained by any of its known actions on vesicular protein or ion channels. Therefore, the exact mechanism(s) underlying the antiepileptic effects of levetiracetam remains uncertain.
- "ROLE OF ΔFOSB IN THE NUCLEUS ACCUMBENS". Mount Sinai School of Medicine. NESTLER LAB: LABORATORY OF MOLECULAR PSYCHIATRY. Archived from the original on 28 June 2017. Retrieved 6 September 2014.
- Furuyashiki T, Deguchi Y (August 2012). "". Brain and Nerve = Shinkei Kenkyū No Shinpo (in Japanese). 64 (8): 919–26. PMID 22868883.
- Nestler EJ (April 2015). "∆FosB: a transcriptional regulator of stress and antidepressant responses". European Journal of Pharmacology. 753: 66–72. doi:10.1016/j.ejphar.2014.10.034. PMC 4380559. PMID 25446562.
In more recent years, prolonged induction of ∆FosB has also been observed within NAc in response to chronic administration of certain forms of stress. Increasing evidence indicates that this induction represents a positive, homeostatic adaptation to chronic stress, since overexpression of ∆FosB in this brain region promotes resilience to stress, whereas blockade of its activity promotes stress susceptibility. Chronic administration of several antidepressant medications also induces ∆FosB in the NAc, and this induction is required for the therapeutic-like actions of these drugs in mouse models. Validation of these rodent findings is the demonstration that depressed humans, examined at autopsy, display reduced levels of ∆FosB within the NAc. As a transcription factor, ΔFosB produces this behavioral phenotype by regulating the expression of specific target genes, which are under current investigation. These studies of ΔFosB are providing new insight into the molecular basis of depression and antidepressant action, which is defining a host of new targets for possible therapeutic development.
- Dietz DM, Kennedy PJ, Sun H, Maze I, Gancarz AM, Vialou V, Koo JW, Mouzon E, Ghose S, Tamminga CA, Nestler EJ (February 2014). "ΔFosB induction in prefrontal cortex by antipsychotic drugs is associated with negative behavioral outcomes". Neuropsychopharmacology. 39 (3): 538–44. doi:10.1038/npp.2013.255. PMC 3895248. PMID 24067299.
Further reading
- Schuermann M, Jooss K, Müller R (April 1991). "fosB is a transforming gene encoding a transcriptional activator". Oncogene. 6 (4): 567–76. PMID 1903195.
- Brown JR, Ye H, Bronson RT, Dikkes P, Greenberg ME (July 1996). "A defect in nurturing in mice lacking the immediate early gene fosB". Cell. 86 (2): 297–309. doi:10.1016/S0092-8674(00)80101-4. PMID 8706134. S2CID 17266171.
- Heximer SP, Cristillo AD, Russell L, Forsdyke DR (December 1996). "Sequence analysis and expression in cultured lymphocytes of the human FOSB gene (G0S3)". DNA and Cell Biology. 15 (12): 1025–38. doi:10.1089/dna.1996.15.1025. PMID 8985116.
- Liberati NT, Datto MB, Frederick JP, Shen X, Wong C, Rougier-Chapman EM, Wang XF (April 1999). "Smads bind directly to the Jun family of AP-1 transcription factors". Proceedings of the National Academy of Sciences of the United States of America. 96 (9): 4844–9. Bibcode:1999PNAS...96.4844L. doi:10.1073/pnas.96.9.4844. PMC 21779. PMID 10220381.
- Yamamura Y, Hua X, Bergelson S, Lodish HF (November 2000). "Critical role of Smads and AP-1 complex in transforming growth factor-beta -dependent apoptosis". The Journal of Biological Chemistry. 275 (46): 36295–302. doi:10.1074/jbc.M006023200. PMID 10942775.
- Bergman MR, Cheng S, Honbo N, Piacentini L, Karliner JS, Lovett DH (February 2003). "A functional activating protein 1 (AP-1) site regulates matrix metalloproteinase 2 (MMP-2) transcription by cardiac cells through interactions with JunB-Fra1 and JunB-FosB heterodimers". Biochemical Journal. 369 (Pt 3): 485–96. doi:10.1042/BJ20020707. PMC 1223099. PMID 12371906.
- Milde-Langosch K, Kappes H, Riethdorf S, Löning T, Bamberger AM (February 2003). "FosB is highly expressed in normal mammary epithelia, but down-regulated in poorly differentiated breast carcinomas". Breast Cancer Research and Treatment. 77 (3): 265–75. doi:10.1023/A:1021887100216. PMID 12602926. S2CID 987857.
- Baumann S, Hess J, Eichhorst ST, Krueger A, Angel P, Krammer PH, Kirchhoff S (March 2003). "An unexpected role for FosB in activation-induced cell death of T cells". Oncogene. 22 (9): 1333–9. doi:10.1038/sj.onc.1206126. PMID 12618758. S2CID 10696422.
- Holmes DI, Zachary I (January 2004). "Placental growth factor induces FosB and c-Fos gene expression via Flt-1 receptors". FEBS Letters. 557 (1–3): 93–8. Bibcode:2004FEBSL.557...93H. doi:10.1016/S0014-5793(03)01452-2. PMID 14741347. S2CID 6596900.
- Konsman JP, Blomqvist A (May 2005). "Forebrain patterns of c-Fos and FosB induction during cancer-associated anorexia-cachexia in rat". The European Journal of Neuroscience. 21 (10): 2752–66. doi:10.1111/j.1460-9568.2005.04102.x. PMID 15926923. S2CID 40045788.
External links
- ROLE OF ΔFOSB IN THE NUCLEUS ACCUMBENS Archived 28 June 2017 at the Wayback Machine
- KEGG Pathway – human alcohol addiction
- KEGG Pathway – human amphetamine addiction
- KEGG Pathway – human cocaine addiction
- FOSB+protein,+human at the U.S. National Library of Medicine Medical Subject Headings (MeSH)
This article incorporates text from the United States National Library of Medicine, which is in the public domain.
Reinforcement disorders: addiction and dependence | |||||||||||
---|---|---|---|---|---|---|---|---|---|---|---|
Addiction |
| ||||||||||
Dependence |
| ||||||||||
Treatment and management |
| ||||||||||
See also | |||||||||||
Transcription factors and intracellular receptors | |||||||||||||||||||||||||||||||
---|---|---|---|---|---|---|---|---|---|---|---|---|---|---|---|---|---|---|---|---|---|---|---|---|---|---|---|---|---|---|---|
| |||||||||||||||||||||||||||||||
| |||||||||||||||||||||||||||||||
| |||||||||||||||||||||||||||||||
| |||||||||||||||||||||||||||||||
| |||||||||||||||||||||||||||||||
see also transcription factor/coregulator deficiencies |
Interactions
Methamphetamine is metabolized by the liver enzyme CYP2D6, so CYP2D6 inhibitors (e.g., selective serotonin reuptake inhibitors (SSRIs)) will prolong the elimination half-life of methamphetamine. Methamphetamine also interacts with monoamine oxidase inhibitors (MAOIs), since both MAOIs and methamphetamine increase plasma catecholamines; therefore, concurrent use of both is dangerous. Methamphetamine may decrease the effects of sedatives and depressants and increase the effects of antidepressants and other stimulants as well. Methamphetamine may counteract the effects of antihypertensives and antipsychotics due to its effects on the cardiovascular system and cognition respectively. The pH of gastrointestinal content and urine affects the absorption and excretion of methamphetamine. Specifically, acidic substances will reduce the absorption of methamphetamine and increase urinary excretion, while alkaline substances do the opposite. Due to the effect pH has on absorption, proton pump inhibitors, which reduce gastric acid, are known to interact with methamphetamine.
Pharmacology

Pharmacodynamics
Methamphetamine has been identified as a potent full agonist of trace amine-associated receptor 1 (TAAR1), a G protein-coupled receptor (GPCR) that regulates brain catecholamine systems. Activation of TAAR1, via adenylyl cyclase, increases cyclic adenosine monophosphate (cAMP) production and either completely inhibits or reverses the transport direction of the dopamine transporter (DAT), norepinephrine transporter (NET), and serotonin transporter (SERT). When methamphetamine binds to TAAR1, it triggers transporter phosphorylation via protein kinase A (PKA) and protein kinase C (PKC) signaling, ultimately resulting in the internalization or reverse function of monoamine transporters. Other transporters that methamphetamine is known to inhibit are vesicular monoamine transporter 1 (VMAT1), vesicular monoamine transporter 2 (VMAT2), SLC22A3, and SLC22A5. SLC22A3 is an extraneuronal monoamine transporter that is present in astrocytes and SLC22A5 is a high-affinity carnitine transporter. When methamphetamine interacts with VMAT2, it induces a release of monoamines from the synaptic vesicles (vesicles that stores monoamines) into the cytosol (intracellular fluid) of the presynaptic neuron.
Methamphetamine is also an agonist of the alpha-2 adrenergic receptors and sigma receptors with a greater affinity for σ1 than σ2, and inhibits vesicular monoamine transporter 1 (VMAT1), monoamine oxidase B (MAO-B), and monoamine oxidase A (MAO-A). Methamphetamine is known to inhibit the CYP2D6 liver enzyme as well. Dextromethamphetamine is a stronger psychostimulant (approximately ten times on striatal dopamine), but levomethamphetamine, with stronger peripheral effects, has a longer half-life and longer perceived effects among addicts. At high doses, both enantiomers of methamphetamine can induce similar stereotypy and methamphetamine psychosis, but shorter psychodynamic effect for levomethamphetamine.
Methamphetamine is a known neurotoxin in both lab animals and humans. Magnetic resonance imaging studies on human methamphetamine users have also found evidence of neurodegeneration, or adverse neuroplastic changes in brain structure and function. In particular, methamphetamine appears to cause hyperintensity and hypertrophy of white matter, marked shrinkage of hippocampi, and reduced gray matter in the cingulate cortex, limbic cortex, and paralimbic cortex in recreational methamphetamine users. Moreover, evidence suggests that adverse changes in the level of biomarkers of metabolic integrity and synthesis occur in recreational users, such as a reduction in N-acetylaspartate and creatine levels and elevated levels of choline and myoinositol.
Comparison to amphetamine pharmacodynamics
Both amphetamine and methamphetamine are potent CNS stimulants with a few biomolecular targets and affected transporters in common; however, there are important pharmacodynamic differences between the two compounds. Both compounds are potent trace amine-associated receptor 1 (TAAR1) agonists (causing non-competitive inhibition of DAT, NET, and SERT) and inhibitors of VMAT2, SLC22A3, and SLC22A5. However, methamphetamine appears to bind at a different site at VMAT2 than amphetamine. Methamphetamine also inhibits VMAT1, has agonist activity at all alpha-2 adrenergic receptor and sigma receptor subtypes, and is directly toxic to dopamine neurons in humans, whereas there is no evidence of acute amphetamine toxicity in humans. Sigma receptor activity is known to potentiate the stimulant and neurotoxic effects of methamphetamine.
In contrast to the adverse neuroplastic effects evident in methamphetamine addicts, long-term use of amphetamine or methylphenidate at therapeutic doses appears to produce beneficial changes in brain function and structure, such as normalization of the right caudate nucleus.
Pharmacokinetics
Following oral administration, methamphetamine is well-absorbed into the bloodstream, with peak plasma methamphetamine concentrations achieved in approximately 3.13–6.3 hours post ingestion. Methamphetamine is also well absorbed following inhalation and following intranasal administration. Due to the high lipophilicity of methamphetamine, it can readily move through the blood–brain barrier faster than other stimulants, where it is more resistant to degradation by monoamine oxidase. The amphetamine metabolite peaks at 10–24 hours. It is excreted by the kidneys, with the rate of excretion into the urine heavily influenced by urinary pH. When taken orally, 30–54% of the dose is excreted in urine as methamphetamine and 10–23% as amphetamine. Following IV doses, about 45% is excreted as methamphetamine and 7% as amphetamine. The half-life of methamphetamine is variable with a mean value of between 5 and 12 hours.
CYP2D6, dopamine β-hydroxylase, flavin-containing monooxygenase, butyrate-CoA ligase, and glycine N-acyltransferase are the enzymes known to metabolize methamphetamine or its metabolites in humans. The primary metabolites are amphetamine and 4-hydroxymethamphetamine; other minor metabolites include: 4-hydroxyamphetamine, 4-hydroxynorephedrine, 4-hydroxyphenylacetone, benzoic acid, hippuric acid, norephedrine, and phenylacetone, the metabolites of amphetamine. Among these metabolites, the active sympathomimetics are amphetamine, 4‑hydroxyamphetamine, 4‑hydroxynorephedrine, 4-hydroxymethamphetamine, and norephedrine.
The main metabolic pathways involve aromatic para-hydroxylation, aliphatic alpha- and beta-hydroxylation, N-oxidation, N-dealkylation, and deamination. The known metabolic pathways include:
Metabolic pathways of methamphetamine
![]() ![]() |
Detection in biological fluids
Methamphetamine and amphetamine are often measured in urine or blood as part of a drug test for sports, employment, poisoning diagnostics, and forensics. Chiral techniques may be employed to help distinguish the source the drug to determine whether it was obtained illicitly or legally via prescription or prodrug. Chiral separation is needed to assess the possible contribution of levomethamphetamine (e.g., Vicks Vapoinhaler) toward a positive test result. Dietary zinc supplements can mask the presence of methamphetamine and other drugs in urine.
Physical and chemical properties

Methamphetamine is a chiral compound with two enantiomers, dextromethamphetamine and levomethamphetamine. At room temperature, the free base of methamphetamine is a clear and colorless liquid with an odor characteristic of geranium leaves. It is soluble in diethyl ether and ethanol as well as miscible with chloroform. In contrast, the methamphetamine hydrochloride salt is odorless with a bitter taste. It has a melting point between 170 to 175 °C (338 to 347 °F) and, at room temperature, occurs as white crystals or a white crystalline powder. The hydrochloride salt is also freely soluble in ethanol and water.
Degradation
Bleach exposure time and concentration are correlated with destruction of methamphetamine. Methamphetamine in soils has shown to be a persistent pollutant. Methamphetamine is largely degraded within 30 days in a study of bioreactors under exposure to light in wastewater.
Synthesis
Racemic methamphetamine may be prepared starting from phenylacetone by either the Leuckart or reductive amination methods. In the Leuckart reaction, one equivalent of phenylacetone is reacted with two equivalents of N-methylformamide to produce the formyl amide of methamphetamine plus carbon dioxide and methylamine as side products. In this reaction, an iminium cation is formed as an intermediate which is reduced by the second equivalent of N-methylformamide. The intermediate formyl amide is then hydrolyzed under acidic aqueous conditions to yield methamphetamine as the final product. Alternatively, phenylacetone can be reacted with methylamine under reducing conditions to yield methamphetamine.
Methamphetamine synthesis

History, society, and culture
Main article: History and culture of substituted amphetamines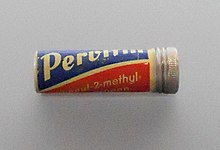
Amphetamine, discovered before methamphetamine, was first synthesized in 1887 in Germany by Romanian chemist Lazăr Edeleanu who named it phenylisopropylamine. Shortly after, methamphetamine was synthesized from ephedrine in 1893 by Japanese chemist Nagai Nagayoshi. Three decades later, in 1919, methamphetamine hydrochloride was synthesized by pharmacologist Akira Ogata via reduction of ephedrine using red phosphorus and iodine.
During World War II, Pervitin (methamphetamine) developed by Berlin-based Temmler pharmaceutical company was used extensively by all branches of the German armed forces (Luftwaffe pilots, in particular) for its performance enhancing stimulant effects and to induce extended wakefulness. Pervitin became colloquially known among the German troops as "Tank-Chocolates" (Panzerschokolade), "Stuka-Tablets" (Stuka-Tabletten) and "Herman-Göring-Pills" (Hermann-Göring-Pillen).
Obetrol, patented by Obetrol Pharmaceuticals in the 1950s and indicated for treatment of obesity, was one of the first brands of pharmaceutical methamphetamine products. Due to the psychological and stimulant effects of methamphetamine, Obetrol became a popular diet pill in America in the 1950s and 1960s. Eventually, as the addictive properties of the drug became known, governments began to strictly regulate the production and distribution of methamphetamine. For example, during the early 1970s in the United States, methamphetamine became a schedule II controlled substance under the Controlled Substances Act. Currently, methamphetamine is sold under the trade name Desoxyn, trademarked by the Danish pharmaceutical company Lundbeck. As of January 2013, the Desoxyn trademark had been sold to Italian pharmaceutical company Recordati.
Present legal status
Main article: Legal status of methamphetamineThe production, distribution, sale, and possession of methamphetamine is restricted or illegal in many jurisdictions. Methamphetamine has been placed in schedule II of the United Nations Convention on Psychotropic Substances treaty.
See also
2Notes
- Image legend
Reference notes
References
- ^ "Enzymes". Methamphetamine. University of Alberta. 8 February 2013. Retrieved 31 December 2013.
{{cite encyclopedia}}
:|work=
ignored (help) Cite error: The named reference "DrugBank Enzymes" was defined multiple times with different content (see the help page). - ^ Cite error: The named reference
Desoxyn
was invoked but never defined (see the help page). - ^ Miller GM (January 2011). "The emerging role of trace amine-associated receptor 1 in the functional regulation of monoamine transporters and dopaminergic activity". J. Neurochem. 116 (2): 164–176. doi:10.1111/j.1471-4159.2010.07109.x. PMC 3005101. PMID 21073468.
- ^ "Targets". Methamphetamine. University of Alberta. 8 February 2013. Retrieved 31 December 2013.
{{cite encyclopedia}}
:|work=
ignored (help) - Borowsky B, Adham N, Jones KA, Raddatz R, Artymyshyn R, Ogozalek KL, Durkin MM, Lakhlani PP, Bonini JA, Pathirana S, Boyle N, Pu X, Kouranova E, Lichtblau H, Ochoa FY, Branchek TA, Gerald C (July 2001). "Trace amines: identification of a family of mammalian G protein-coupled receptors". Proc. Natl. Acad. Sci. U.S.A. 98 (16): 8966–8971. doi:10.1073/pnas.151105198. PMC 55357. PMID 11459929.
{{cite journal}}
: CS1 maint: multiple names: authors list (link) - Xie Z, Miller GM (July 2009). "A receptor mechanism for methamphetamine action in dopamine transporter regulation in brain". J. Pharmacol. Exp. Ther. 330 (1): 316–325. doi:10.1124/jpet.109.153775. PMC 2700171. PMID 19364908.
- ^ "Transporters". Methamphetamine. University of Alberta. 8 February 2013. Retrieved 31 December 2013.
{{cite encyclopedia}}
:|work=
ignored (help) - Inazu M, Takeda H, Matsumiya T (August 2003). "". Nihon Shinkei Seishin Yakurigaku Zasshi (in Japanese). 23 (4): 171–178. PMID 13677912.
{{cite journal}}
: CS1 maint: multiple names: authors list (link) - ^ Eiden LE, Weihe E (January 2011). "VMAT2: a dynamic regulator of brain monoaminergic neuronal function interacting with drugs of abuse". Ann. N. Y. Acad. Sci. 1216: 86–98. doi:10.1111/j.1749-6632.2010.05906.x. PMID 21272013.
- ^ Kaushal N, Matsumoto RR (March 2011). "Role of sigma receptors in methamphetamine-induced neurotoxicity". Curr Neuropharmacol. 9 (1): 54–57. doi:10.2174/157015911795016930. PMC 3137201. PMID 21886562.
- ^ Rodvelt KR, Miller DK (September 2010). "Could sigma receptor ligands be a treatment for methamphetamine addiction?". Curr Drug Abuse Rev. 3 (3): 156–162. doi:10.2174/1874473711003030156. PMID 21054260.
- Melega WP, Cho AK, Schmitz D, Kuczenski R, Segal DS (February 1999). "l-methamphetamine pharmacokinetics and pharmacodynamics for assessment of in vivo deprenyl-derived l-methamphetamine". J. Pharmacol. Exp. Ther. 288 (2): 752–758. PMID 9918585.
{{cite journal}}
: CS1 maint: multiple names: authors list (link) - ^ Kuczenski R, Segal DS, Cho AK, Melega W (February 1995). "Hippocampus norepinephrine, caudate dopamine and serotonin, and behavioral responses to the stereoisomers of amphetamine and methamphetamine". J. Neurosci. 15 (2): 1308–1317. PMID 7869099.
{{cite journal}}
: CS1 maint: multiple names: authors list (link) - ^ Mendelson J, Uemura N, Harris D, Nath RP, Fernandez E, Jacob P, Everhart ET, Jones RT (October 2006). "Human pharmacology of the methamphetamine stereoisomers". Clin. Pharmacol. Ther. 80 (4): 403–420. doi:10.1016/j.clpt.2006.06.013. PMID 17015058.
{{cite journal}}
: CS1 maint: multiple names: authors list (link) - ^ Cite error: The named reference
Malenka
was invoked but never defined (see the help page). - ^ Cite error: The named reference
pmid19328213
was invoked but never defined (see the help page). - Itzhak Y, Martin JL, Ali SF (October 2002). "Methamphetamine-induced dopaminergic neurotoxicity in mice: long-lasting sensitization to the locomotor stimulation and desensitization to the rewarding effects of methamphetamine". Progress in Neuro-psychopharmacology & Biological Psychiatry. 26 (6): 1177–1183. doi:10.1016/S0278-5846(02)00257-9. PMID 12452543.
{{cite journal}}
: CS1 maint: multiple names: authors list (link) - Davidson C, Gow AJ, Lee TH, Ellinwood EH (August 2001). "Methamphetamine neurotoxicity: necrotic and apoptotic mechanisms and relevance to human abuse and treatment". Brain Research. Brain Research Reviews. 36 (1): 1–22. doi:10.1016/S0165-0173(01)00054-6. PMID 11516769.
{{cite journal}}
: CS1 maint: multiple names: authors list (link) - ^ "Transporters". Amphetamine. University of Alberta. 8 February 2013. Retrieved 13 October 2013.
{{cite encyclopedia}}
:|work=
ignored (help) - "Targets". Amphetamine. University of Alberta. 8 February 2013. Retrieved 13 October 2013.
{{cite encyclopedia}}
:|work=
ignored (help) - Amphetamine. National Center for Biotechnology Information. Retrieved 13 October 2013.
{{cite encyclopedia}}
:|work=
ignored (help) - Sulzer D, Sonders MS, Poulsen NW, Galli A (April 2005). "Mechanisms of neurotransmitter release by amphetamines: a review". Prog. Neurobiol. 75 (6): 406–433. doi:10.1016/j.pneurobio.2005.04.003. PMID 15955613.
They also demonstrated competition for binding between METH and reserpine, suggesting they might bind to the same site on VMAT. George Uhl's laboratory similarly reported that AMPH displaced the VMAT2 blocker tetrabenazine (Gonzalez et al., 1994). It should be noted that tetrabenazine and reserpine are thought to bind to different sites on VMAT (Schuldiner et al., 1993a)
{{cite journal}}
: CS1 maint: multiple names: authors list (link) - Hart H, Radua J, Nakao T, Mataix-Cols D, Rubia K (February 2013). "Meta-analysis of functional magnetic resonance imaging studies of inhibition and attention in attention-deficit/hyperactivity disorder: exploring task-specific, stimulant medication, and age effects". JAMA Psychiatry. 70 (2): 185–198. doi:10.1001/jamapsychiatry.2013.277. PMID 23247506.
{{cite journal}}
: CS1 maint: multiple names: authors list (link) - Spencer TJ, Brown A, Seidman LJ, Valera EM, Makris N, Lomedico A, Faraone SV, Biederman J (September 2013). "Effect of psychostimulants on brain structure and function in ADHD: a qualitative literature review of magnetic resonance imaging-based neuroimaging studies". J. Clin. Psychiatry. 74 (9): 902–917. doi:10.4088/JCP.12r08287. PMC 3801446. PMID 24107764.
{{cite journal}}
: CS1 maint: multiple names: authors list (link) - ^ "Pharmacology". Methamphetamine. University of Alberta. 8 February 2013. Retrieved 31 December 2013.
{{cite encyclopedia}}
:|work=
ignored (help) - ^ Schep LJ, Slaughter RJ, Beasley DM (August 2010). "The clinical toxicology of metamfetamine". Clinical Toxicology (Philadelphia, Pa.). 48 (7): 675–694. doi:10.3109/15563650.2010.516752. ISSN 1556-3650. PMID 20849327.
{{cite journal}}
: CS1 maint: multiple names: authors list (link) - Cite error: The named reference
DBH ref
was invoked but never defined (see the help page). - Cite error: The named reference
FMO
was invoked but never defined (see the help page). - "Substrate/Product". butyrate-CoA ligase. Technische Universität Braunschweig. Retrieved 7 May 2014.
{{cite encyclopedia}}
:|work=
ignored (help) - "Substrate/Product". glycine N-acyltransferase. Technische Universität Braunschweig. Retrieved 7 May 2014.
{{cite encyclopedia}}
:|work=
ignored (help) - ^ "Adderall XR Prescribing Information" (PDF). United States Food and Drug Administration. December 2013. pp. 12–13. Retrieved 30 December 2013.
- ^ Amphetamine. National Center for Biotechnology Information. Retrieved 12 October 2013.
{{cite encyclopedia}}
:|work=
ignored (help) - ^ Santagati NA, Ferrara G, Marrazzo A, Ronsisvalle G (September 2002). "Simultaneous determination of amphetamine and one of its metabolites by HPLC with electrochemical detection". J. Pharm. Biomed. Anal. 30 (2): 247–255. doi:10.1016/S0731-7085(02)00330-8. PMID 12191709.
{{cite journal}}
: CS1 maint: multiple names: authors list (link) - "Compound Summary". p-Hydroxyamphetamine. National Center for Biotechnology Information. Retrieved 15 October 2013.
{{cite encyclopedia}}
:|work=
ignored (help) - "Compound Summary". p-Hydroxynorephedrine. National Center for Biotechnology Information. Retrieved 15 October 2013.
{{cite encyclopedia}}
:|work=
ignored (help) - "Compound Summary". Phenylpropanolamine. National Center for Biotechnology Information. Retrieved 15 October 2013.
{{cite encyclopedia}}
:|work=
ignored (help) - Liddle DG, Connor DJ (June 2013). "Nutritional supplements and ergogenic AIDS". Prim. Care. 40 (2): 487–505. doi:10.1016/j.pop.2013.02.009. PMID 23668655.
- Kraemer T, Maurer HH (August 1998). "Determination of amphetamine, methamphetamine and amphetamine-derived designer drugs or medicaments in blood and urine". J. Chromatogr. B Biomed. Sci. Appl. 713 (1): 163–187. doi:10.1016/S0378-4347(97)00515-X. PMID 9700558.
- Kraemer T, Paul LD (August 2007). "Bioanalytical procedures for determination of drugs of abuse in blood". Anal. Bioanal. Chem. 388 (7): 1415–1435. doi:10.1007/s00216-007-1271-6. PMID 17468860.
- Goldberger BA, Cone EJ (July 1994). "Confirmatory tests for drugs in the workplace by gas chromatography-mass spectrometry". J. Chromatogr. A. 674 (1–2): 73–86. doi:10.1016/0021-9673(94)85218-9. PMID 8075776.
- ^ Paul BD, Jemionek J, Lesser D, Jacobs A, Searles DA (September 2004). "Enantiomeric separation and quantitation of (+/-)-amphetamine, (+/-)-methamphetamine, (+/-)-MDA, (+/-)-MDMA, and (+/-)-MDEA in urine specimens by GC-EI-MS after derivatization with (R)-(−)- or (S)-(+)-alpha-methoxy-alpha-(trifluoromethy)phenylacetyl chloride (MTPA)". J Anal Toxicol. 28 (6): 449–455. doi:10.1093/jat/28.6.449. PMID 15516295.
{{cite journal}}
: CS1 maint: multiple names: authors list (link) - de la Torre R, Farré M, Navarro M, Pacifici R, Zuccaro P, Pichini S (2004). "Clinical pharmacokinetics of amfetamine and related substances: monitoring in conventional and non-conventional matrices". Clin Pharmacokinet. 43 (3): 157–185. doi:10.2165/00003088-200443030-00002. PMID 14871155.
{{cite journal}}
: CS1 maint: multiple names: authors list (link) - Baselt RC (2011). Disposition of toxic drugs and chemicals in man. Seal Beach, Ca.: Biomedical Publications. pp. 1027–1030. ISBN 0-9626523-8-5.
- Venkatratnam A, Lents NH (July 2011). "Zinc reduces the detection of cocaine, methamphetamine, and THC by ELISA urine testing". J. Anal. Toxicol. 35 (6): 333–340. doi:10.1093/anatox/35.6.333. PMID 21740689.
- ^ Cite error: The named reference
Pubchem2
was invoked but never defined (see the help page). - Nakayama, MT. "Chemical Interaction of Bleach and Methamphetamine: A Study of Degradation and Transformation Effects". gradworks. UNIVERSITY OF CALIFORNIA, DAVIS. Retrieved 17 October 2014.
- Pal R, Megharaj M, Kirkbride KP, Heinrich T, Naidu R (October 2011). "Biotic and abiotic degradation of illicit drugs, their precursor, and by-products in soil". Chemosphere. 85 (6): 1002–9. doi:10.1016/j.chemosphere.2011.06.102. PMID 21777940.
{{cite journal}}
: CS1 maint: multiple names: authors list (link) - Bagnall J, Malia L, Lubben A, Kasprzyk-Hordern B (October 2013). "Stereoselective biodegradation of amphetamine and methamphetamine in river microcosms". Water Res. 47 (15): 5708–18. doi:10.1016/j.watres.2013.06.057. PMID 23886544.
{{cite journal}}
: CS1 maint: multiple names: authors list (link) - Crossley FS, Moore ML (November 1944). "Studies on the Leuckart reaction". The Journal of Organic Chemistry. 9 (6): 529–536. doi:10.1021/jo01188a006.
- ^ Kunalan V, Nic Daéid N, Kerr WJ, Buchanan HA, McPherson AR (September 2009). "Characterization of route specific impurities found in methamphetamine synthesized by the Leuckart and reductive amination methods". Anal. Chem. 81 (17): 7342–7348. doi:10.1021/ac9005588. PMC 3662403. PMID 19637924.
{{cite journal}}
: CS1 maint: multiple names: authors list (link) - Rassool GH (2009). Alcohol and Drug Misuse: A Handbook for Students and Health Professionals. London: Routledge. p. 113. ISBN 978-0-203-87117-1.
- ^ "Historical overview of methamphetamine". Vermont Department of Health. Government of Vermont. Retrieved 29 January 2012.
- Grobler, Sias R.; Chikte, Usuf; Westraat, Jaco (2011). "The pH Levels of Different Methamphetamine Drug Samples on the Street Market in Cape Town". ISRN Dentistry. 2011: 1–4. doi:10.5402/2011/974768. PMC 3189445. PMID 21991491.
{{cite journal}}
: CS1 maint: unflagged free DOI (link) - "Historical overview of methamphetamine". Vermont Department of Health. Retrieved January 2012.
{{cite web}}
: Check date values in:|accessdate=
(help) - "The Nazi Death Machine: Hitler's Drugged Soldiers". Der Spiegel, 6 May 2005.
- Defalque RJ, Wright AJ (April 2011). "Methamphetamine for Hitler's Germany: 1937 to 1945". Bull. Anesth. Hist. 29 (2): 21–24, 32. PMID 22849208.
- ^ Rasmussen, Nicolas (March 2008). On Speed: The Many Lives of Amphetamine (1 ed.). New York University Press. p. 148. ISBN 0-8147-7601-9.
- "Controlled Substances Act". United States Food and Drug Administration. 11 June 2009. Retrieved 4 November 2013.
- "Desoxyn". Lundbeck: Desoxyn. Retrieved December 2012.
{{cite web}}
: Check date values in:|accessdate=
(help) - "Recordati: Desoxyn". Recordati SP. Retrieved May 2013.
{{cite web}}
: Check date values in:|accessdate=
(help) - United Nations Office on Drugs and Crime (2007). Preventing Amphetamine-type Stimulant Use Among Young People: A Policy and Programming Guide (PDF). New York: United Nations. ISBN 978-92-1-148223-2. Retrieved 11 November 2013.
- ^ "List of psychotropic substances under international control" (PDF). International Narcotics Control Board. United Nations. August 2003. Archived from the original (PDF) on 5 December 2005. Retrieved 19 November 2005.
External links
Amphetamine | |||||||||
---|---|---|---|---|---|---|---|---|---|
Main articles and pharmaceuticals |
| ||||||||
Neuropharmacology |
| ||||||||
Active metabolites | |||||||||
Related articles |
| ||||||||
Recreational drug use | |||||||||||||||||||||||||||
---|---|---|---|---|---|---|---|---|---|---|---|---|---|---|---|---|---|---|---|---|---|---|---|---|---|---|---|
| |||||||||||||||||||||||||||
| |||||||||||||||||||||||||||
| |||||||||||||||||||||||||||
|
Methamphetamine | |||||
---|---|---|---|---|---|
Enantiomers | |||||
Neuropharmacology |
| ||||
Health | |||||
History and culture | |||||
Law | |||||
Ethnicity and nationality |
ADHD pharmacotherapies | |
---|---|
CNSTooltip central nervous system stimulants | |
Non-classical CNS stimulants | |
α2-adrenoceptor agonists | |
Antidepressants | |
Miscellaneous/others | |
Related articles |
|
Trace amine-associated receptor modulators | |||||||||||
---|---|---|---|---|---|---|---|---|---|---|---|
TAAR1Tooltip Trace amine-associated receptor 1 |
| ||||||||||
TAAR5Tooltip Trace amine-associated receptor 5 |
| ||||||||||
Notes: (1) TAAR1 activity of ligands varies significantly between species. Some agents that are TAAR1 ligands in some species are not in other species. This navbox includes all TAAR1 ligands regardless of species. (2) See the individual pages for references, as well as the List of trace amines, TAAR, and TAAR1 pages. See also: Receptor/signaling modulators |
Phenethylamines | |
---|---|
Phenethylamines |
|
Amphetamines |
|
Phentermines |
|
Cathinones |
|
Phenylisobutylamines | |
Phenylalkylpyrrolidines | |
Catecholamines (and close relatives) |
|
Miscellaneous |
|
- Human genes
- Addiction
- Oncogenes
- Transcription factors
- Methamphetamine
- Amphetamine
- Anorectics
- Cardiac stimulants
- Euphoriants
- Japanese inventions
- Management of obesity
- Norepinephrine-dopamine releasing agents
- Phenethylamines
- Sigma agonists
- Stimulants
- Substituted amphetamines
- Sympathomimetics
- TAAR1 agonists
- Treatment and management of attention deficit hyperactivity disorder
- VMAT inhibitors